Premium Only Content
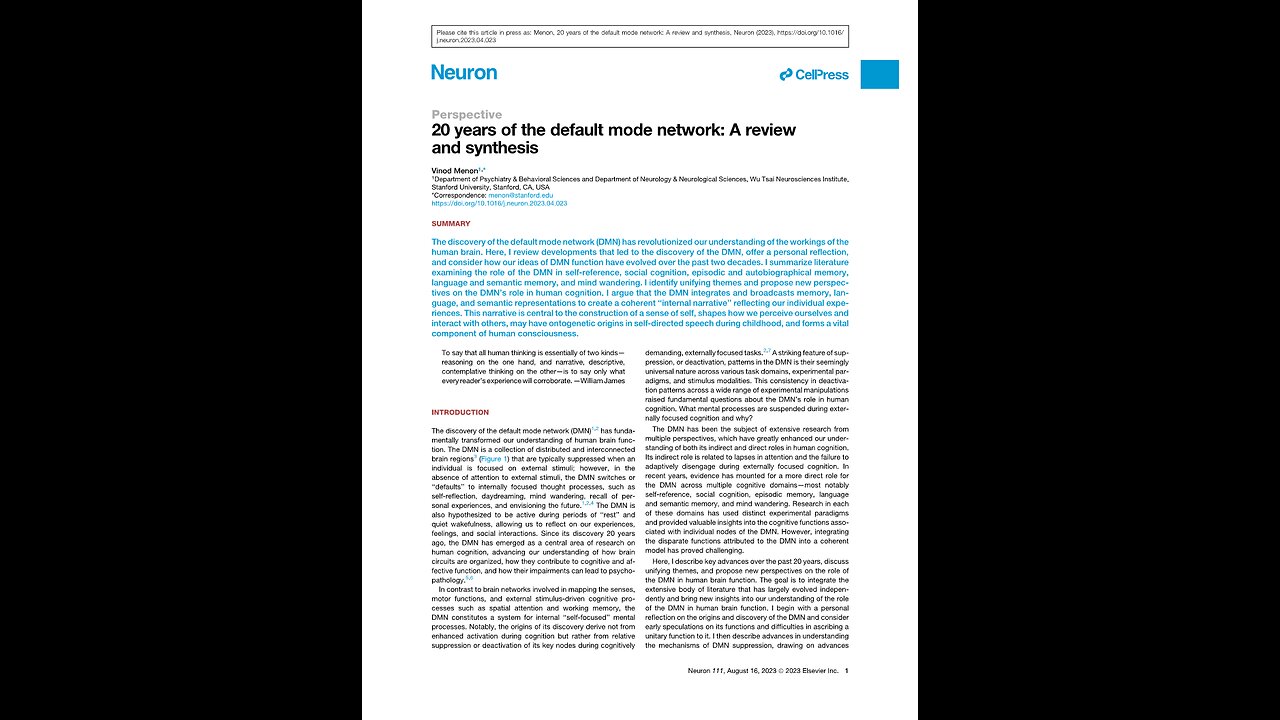
The default mode network. Vinod Menon, A Puke(TM) Audiopaper.
Index of Other Science Articles:
https://rumble.com/v3t4yzj-index-of-science.-music-by-dan-vasc.html
20 years of the default mode network: A review and synthesis.
Vinod Menon, Stanford University, Stanford, CA, USA.
menon@stanford.edu
Summary.
The discovery of the default mode network (DMN) has revolutionized our understanding of the workings of the human brain. Here, I review developments that led to the discovery of the DMN, offer a personal reflection, and consider how our ideas of DMN function have evolved over the past two decades. I summarize literature examining the role of the DMN in self-reference, social cognition, episodic and autobiographical memory, language and semantic memory, and mind wandering. I identify unifying themes and propose new perspectives on the DMN’s role in human cognition. I argue that the DMN integrates and broadcasts memory, language, and semantic representations to create a coherent internal narrative reflecting our individual experiences. This narrative is central to the construction of a sense of self, shapes how we perceive ourselves and interact with others, may have ontogenetic origins in self-directed speech during childhood, and forms a vital component of human consciousness.
To say that all human thinking is essentially of two kinds, reasoning on the one hand, and narrative, descriptive, contemplative thinking on the other, is to say only what every reader’s experience will corroborate. William James.
Introduction.
The discovery of the default mode network (DMN) has fundamentally transformed our understanding of human brain function. The DMN is a collection of distributed and interconnected brain regions, see Figure 1, that are typically suppressed when an individual is focused on external stimuli, however, in the absence of attention to external stimuli, the DMN switches or defaults to internally focused thought processes, such as self-reflection, daydreaming, mind wandering, recall of personal experiences, and envisioning the future. The DMN is also hypothesized to be active during periods of rest and quiet wakefulness, allowing us to reflect on our experiences, feelings, and social interactions. Since its discovery 20 years ago, the DMN has emerged as a central area of research on human cognition, advancing our understanding of how brain circuits are organized, how they contribute to cognitive and affective function, and how their impairments can lead to psychopathology. In contrast to brain networks involved in mapping the senses, motor functions, and external stimulus-driven cognitive processes such as spatial attention and working memory, the DMN constitutes a system for internal self-focused mental processes. Notably, the origins of its discovery derive not from enhanced activation during cognition but rather from relative suppression or deactivation of its key nodes during cognitively demanding, externally focused tasks. A striking feature of suppression, or deactivation, patterns in the DMN is their seemingly universal nature across various task domains, experimental paradigms, and stimulus modalities. This consistency in deactivation patterns across a wide range of experimental manipulations raised fundamental questions about the DMN’s role in human cognition. What mental processes are suspended during externally focused cognition and why? The DMN has been the subject of extensive research from multiple perspectives, which have greatly enhanced our understanding of both its indirect and direct roles in human cognition. Its indirect role is related to lapses in attention and the failure to adaptively disengage during externally focused cognition. In recent years, evidence has mounted for a more direct role for the DMN across multiple cognitive domains, most notably self-reference, social cognition, episodic memory, language and semantic memory, and mind wandering. Research in each of these domains has used distinct experimental paradigms and provided valuable insights into the cognitive functions associated with individual nodes of the DMN. However, integrating the disparate functions attributed to the DMN into a coherent model has proved challenging.
Here, I describe key advances over the past 20 years, discuss unifying themes, and propose new perspectives on the role of the DMN in human brain function. The goal is to integrate the extensive body of literature that has largely evolved independently and bring new insights into our understanding of the role of the DMN in human brain function. I begin with a personal reflection on the origins and discovery of the DMN and consider early speculations on its functions and difficulties in ascribing a unitary function to it. I then describe advances in understanding the mechanisms of DMN suppression, drawing on advances computational modeling and optogenetic studies with concurrent brain imaging.
Figure 1. Cortical and subcortical nodes of the DMN.
(A) Illustration of DMN nodes as a functionally and anatomically interconnected system.
(B and C) Cortical nodes of the DMN: posterior cingulate cortex (PCC) and retrosplenial cortex (RSC) in posterior medial parietal cortex. Medial PFC (mPFC) with its dorsomedial (dmPFC) and ventromedial (dmPFC) subdivisions, anterior temporal cortex (ATC), middle temporal gyrus (MTG) in lateral temporal cortex, medial temporal lobe (MTL). And angular gyrus (AG) in lateral parietal cortex.
(D) Subcortical nodes of the DMN: anterior and mediodorsal thalamic nuclei, medial septal nuclei, and nucleus accumbens.
The DMN does not operate in isolation, and its functions are shaped by dynamic interactions with other brain networks. I illustrate how understanding these interactions is crucial for a more comprehensive understanding of the DMN’s dynamic role in cognition. I summarize advances in five key cognitive functions now widely associated with the DMN: self-reference, social cognition, episodic memory, language and semantic memory, and mind wandering. I describe unifying themes and perspectives on DMN function and argue that it plays an essential role in integrating memory, language, and semantic representations to create a coherent ‘‘internal narrative’’ in the context of our individual experiences and social interactions. I conclude by highlighting ongoing challenges in the study of DMN function and highlight potential areas for future research.
The DMN, origins and discovery, a personal reflection.
The origins of the discovery of the DMN lie in the surprising finding that a common set of brain areas is suppressed during attention to external stimuli. In 1997, Shulman and colleagues published a meta-analysis summarizing findings across multiple positron emission tomography (PET) datasets they had acquired since the late 1980s. Participants were asked to perform a range of tasks involving manipulations of attention, memory, and language with active, passive, and fixation conditions. Across nine studies of human visual information processing, they examined the consistency of decreased blood flow between experiments during active tasks relative to passive viewing of the same stimulus array.
Figure 2. Key findings leading to discovery of the DMN.
(A) Blood flow decreases in active task minus passive control scans across nine PET functional brain imaging studies. Numbers indicate the 14 brain areas that showed decreased activity when participants processed a visual image in an active task, contrasted with passive viewing in the control state.
(B) Maps of the fraction of oxygen extracted by the brain, showing increases in visual cortex bilaterally. Despite an almost 4-fold difference in blood flow and oxygen consumption between gray and white matter, the fraction of oxygen extracted is relatively uniform, emphasizing the close matching of blood flow and oxygen consumption in the resting, awake brain.
(C) Intrinsic functional connectivity of the PCC, peak blue arrow. Eight significant clusters labeled A to H encompass AG, mPFC, MTG, and MTL.
(D) Intrinsic functional connectivity of the vACC, peak at blue arrow. Four significant clusters labeled A–D encompass dorsal PCC, precuneus, rostral PCC, nucleus accumbens, and hypothalamus, rostral midbrain.
(E) PCC connectivity patterns during low-level visual processing and the resting-state display similar features.
(F) PCC fluctuations are inversely correlated with ventrolateral and dorsolateral PFC.
The areas of the brain that showed consistent decreases during active tasks included the posterior gulate, precuneus (Brodmann area, BA, 31-7). Left inferior parietal cortex (BA 39-40), left dorsolateral frontal cortex (BA 9), left lateral inferior frontal cortex (BA 10-47). Left inferior temporal gyrus (BA 20), medial prefrontal cortex (mPFC) (BA’s 8, 9, 10, and 32), and the right amygdala (Figure 2A).
A subsequent PET study by Mazoyer and colleagues confirmed these findings, the commonality of deactivations led the authors to suggest that brain activity during a conscious resting state may be sustained by a large-scale network of heteromodal associative parietal and frontal cortical areas.
In a pioneering study published in 2001, Raichle and colleagues investigated whether the commonly deactivated regions observed in PET studies might display regionally enhanced activity at rest. They used quantitative metabolic and circulatory measurements to determine whether task-related activity decreases arose from activations in the resting state. However, as they noted, areas of activation were conspicuous by their absence. Surprisingly, all significant deviations from the mean oxygen extraction fraction were deactivations and were observed almost exclusively in the visual system (Figure 2B). Most of the areas of the brain originally identified as deactivations in-task-based PET and fMRI studies were however missing here. Thus, task-related deactivations did not appear to arise from enhanced activations in the resting state, and evidence that deactivated regions may be tonically active in the baseline state proved elusive. A crucial aspect of the study was the framing of a central question in the investigation of human brain function, which led the authors to coin the term default mode. This terminology has proved to be remarkably prescient and insightful and has become widely adopted in the field of neuro science. Like many influential studies, this work raised more important questions than it answered. Was the "default mode" an artifact of baseline conditions used in brain imaging studies? Did the brain regions that showed deactivations in PET studies constitute a coherent network of interconnected nodes? What were the cognitive functions of the default mode, and how could they be investigated directly with experimental task manipulations?
My interest in the putative human DMN began in 1997 while conducting fMRI studies in clinical populations. This interest was sparked by the observation of shared deactivation patterns in a diverse range of experiments, including n-back working memory, auditory and visual attention, and self-paced motor sequencing tasks in both healthy individuals and schizophrenia patients. It was striking that brain areas that showed increased responses with cognitive load differed depending on the task and cognitive domain, but areas with suppressed brain response remained consistent. The deactivation patterns observed in fMRI were similar to those found in PET studies and furthermore, were easily identified at the individual level. Despite this, task-related deactivations were frequently over looked and excluded from further analysis, typical of the fMRI research at that time. This should not be surprising, as in most cases, cognitive and psychological interpretations of the observed deactivation patterns were speculative at best.
During the initial years, my understanding of DMN function was influenced by research on stimulus-independent thoughts in patients with schizophrenia experiencing distress. I vividly recall conversations with a Vietnam war veteran who reported hearing his father’s voice admonishing him while I was scanning him at the Palo Alto VA Medical Center. Subjective experience suggests that we are continually engaged in unconstrained, stimulus-independent thoughts even when no external stimuli are being processed. Studies in patients with psychiatric disorders had suggested that dysfunction of such thoughts was key to understanding symptoms such as hallucinations and depression. We noted, however, that the neural substrates of these subjective processes have not been particularly amenable to experimental analysis as initial attempts using self paced and external stimulus-driven actions proved inconclusive, V M et al, unpublished data.
A different approach to the problem proved more tractable. In 1995, Biswal et al reported that sensorimotor regions engaged during a finger movement task also showed synchronized temporal responses in spontaneous low-frequency, less than 0.1 Hertz, fluctuations. Two observations stood out: correlations in low frequency fluctuations could be used to probe functional brain networks, and these fluctuations reflected coactivation of task relevant regions. During this time, at Stanford, we began to acquire whole-brain resting-state fMRI in healthy adult controls and in patients with depression or Alzheimer’s disease. While data for disorder-related projects were being acquired, Michael Greicius and I decided to examine brain networks associated with working memory task-related deactivations in healthy controls. A key goal was to link task-related deactivations with functional connectivity analysis. We identified brain areas that showed consistent deactivation and examined whether they formed a coherent intrinsic network and how this network was modulated during cognitive processing. The posterior cingulate cortex (PCC) emerged as a natural seed target, based on common loci of task-related deactivations observed in fMRI studies of cognition in healthy adults, on the one hand, and functional impairments observed in PET studies in Alzheimer’s disease on the other. We defined PCC and mPFC regions that showed decreased activity during a working memory task and then examined their functional connectivity during rest. Our analysis revealed that the PCC was strongly coupled with mPFC and several other brain regions that were consistently reported to be deactivated in PET and fMRI studies of cognition, Figures 2C to 2F.
Next, we examined the functional connectivity of PCC and mPFC during a visual perception task and showed that the resulting connectivity maps were nearly identical to those obtained during rest. We argued that although the DMN is thought to be most active during the resting state, it may also persist during passive sensory processing states. Finally, we isolated lateral prefrontal regions that showed increased activity during working memory and examined their connectivity in the resting state. We reported significant negative correlations between the PCC and multiple lateral prefrontal regions, suggesting a mechanism to attenuate network activity in the DMN during cognitive processing. This study constituted the first analysis of the intrinsic, as well as task-related, connectivity of the DMN and provided compelling evidence for the existence of a cohesive network linking brain areas that showed consistent patterns of deactivation across tasks. In retrospect, it is noteworthy that our study made a serious effort to investigate the relationship between the DMN and the lateral PFC and parietal areas activated during high-level cognition. This proved essential in establishing a correspondence between task-related deactivation and intrinsic functional circuits and more broadly between spontaneous neural activity and cognition.
In 2002, we first presented this work at the Organization of Human Brain Mapping in Sendai. The existence of a cohesive, temporally coupled, network of distributed brain areas associated with high-level cognition garnered quite some interest, and findings were subsequently published in PNAS. Building on Raichle and colleagues’ notion of a default mode, Michael Greicius and I coined the phrase default mode network, and since its publication, the DMN has been the focus of over 8,000 studies.
Early speculations on DMN function and challenges in ascribing a unitary function.
Early descriptions of the functional role of DMN nodes individually were speculative at best, and the notion that these nodes formed a network was nonexistent. However, the Jamesian notion of streams of consciousness, that the brain is constantly processing various types of information and experiences, and that these mental processes occur continuously and in parallel, loomed large. In 1997, Shulman and colleagues speculated that increased activity during the passive condition could reflect ongoing processes, such as unconstrained verbally mediated thoughts and monitoring of the external environment, body, and emotional state. In 2001, Raichle and colleagues extended this view further and remarked that the mPFC and PCC may well be the sentinels that monitor our behaviors and streams of consciousness.
In an earlier groundbreaking 1995 study, Andreasen and colleagues concentrated on the same regions from an episodic memory perspective. They examined spontaneous, uncensored thinking about experiences and found that the PCC, restrosplenial cortex (RSC), precuneus, and mPFC demonstrated activation levels comparable to those observed during focused autobiographical episodic memory recall. They proposed that the commonality might indicate active retrieval of past experiences and planning the future. This remarkable early study offered empirical evidence that anticipated much of the field’s subsequent evolution, highlighting the DMN’s involvement in episodic memory. In the same vein, based on analysis of semantic and language tasks, Binder and colleagues argued for DMN involvement in semantic knowledge retrieval during the conscious resting state.
In discussing our discovery of the DMN in 2003, we drew on the close correspondence between deactivations in the PCC, RSC, and hippocampus, and memory systems impacted in Alzheimer’s disease.
We wrote:
Although the precise mental processes supported by the DMN remain to be elucidated, we suggest that the retrieval and manipulation of episodic memories and semantic knowledge are likely candidates.
Beyond these notions, it was clear that, based on what these pioneering studies achieved and what they could not achieve, characterization of the cognitive functions of the DMN would be challenging. First, if the functions of individual DMN nodes were suspended during specific goal-directed behaviors, reading their internal contents would be challenging, as experimental paradigms are typically designed to exclude introspective processes. Second, it was unclear how to develop experimental paradigms that effectively activate DMN nodes above their resting baselines to uncover their essential functions. The cognitive terms attributed to DMN functioning, for example, stimulus-independent thoughts, mind wandering, self-referential processing, were disconnected from traditional fMRI study paradigms. Third, human neuroscience has historically relied heavily on parallels with animal models, but, at that time, there was no known equivalent of the DMN in animals. Moreover, if DMN functions were indeed related to introspection, the self, and autobiographical memory, then the underlying neural mechanisms would be difficult to investigate in animals.
Brain imaging studies in patients with psychiatric and neurological disorders offered new perspectives about DMN function. In quick succession after the discovery of the network, DMN dysfunction was established in patients with Alzheimer’s disease, depression, and schizophrenia. DMN dysfunction rapidly became an important feature of virtually all psychiatric and neurological disorders. Furthermore, pathology differentially affected individual DMN nodes. Notably, DMN dysfunction in Alzheimer’s disease, associated with memory loss, mostly affected the PCC, RSC, and medial temporal lobe (MTL), whereas dysfunction in depression, associated with rumination, mainly affected the ventral mPFC and adjoining rostral, subgenual cingulate cortex. These early clinical studies were clear indications that the contributions of DMN nodes were not unitary and could be dissociated. Thus, neuroimaging studies in clinical groups provided complementary information for expanding our understanding of DMN function. They also exposed the limitations of assigning a unitary function to the DMN.
Indirect role of the DMN in cognition: mechanisms of suppression and cross-network interactions.
The DMN has been implicated in momentary lapses in attention that impair goal-directed behavior. An important early study showed that ineffective suppression of DMN responses impaired selective attention.
Figure 3. Mechanisms of DMN suppression and network switching.
(A) The triple-network model posits a central role for the salience network in processing behaviorally relevant external events leading to suppression of the DMN and its altered dynamic temporal interactions with the salience and frontoparietal networks. The ensuing engagement of cognitive control circuit dynamics and network switching shapes DMN function and its indirect role in cognition.
(B) Onset latencies of event-related responses in six key cortical nodes of the DMN (yellow bars), salience network (blue bars), and frontoparietal network (green bars) during auditory event segmentation. The right AI and adjoining frontal cortex (labeled rFIC) nodes of the salience network display onsets significantly earlier than frontoparietal and DMN nodes. Adapted from Sridharan et al.
(C and D) Net causal outflow (out-in degree) of DMN and salience and frontoparietal networks revealed that the rFIC has a significantly higher net causal outflow than DMN nodes across auditory and visual attention tasks.
By investigating trial-by-trial relationships between brain activity and response time, it was found that less efficient stimulus processing during attentional lapses was characterized by reduced deactivation in the PCC, precuneus, and angular gyrus (AG) nodes of the DMN, along with reduced stimulus-evoked sensory activity and hyperactivity in widespread regions of frontal and parietal cortex. Subsequent research has confirmed this finding and provided converging evidence that DMN suppression was necessary for adaptive disengagement during externally focused cognition. At the very least, these findings suggested an indirect role in cognition through interaction with other brain networks.
An important challenge then was to identify the mechanisms by which the DMN could be suppressed during external stimulus-driven cognition. Two other large-scale brain networks that are directly involved in cognitive control have received considerable attention in this context: the salience network anchored in the anterior insula and dorsal anterior cingulate cortex, with prominent subcortical nodes in the affect and reward processing regions, and the frontoparietal network, anchored in the dorsolateral prefrontal cortex and posterior parietal cortex, Figure 3. Analysis of multiple tasks, using dynamic causal modeling, has demonstrated that the salience network plays an important role in network switching between context-dependent engagement and disengagement of the DMN. The anterior insula node of the salience network, in particular, is crucial for bottom-up detection of salient events and switching between other large-scale networks to facilitate access to attention and working memory resources. Across a wide range of tasks, the anterior insula has emerged as a causal signaling hub that facilitates engagement of the lateral frontoparietal cortex involved in attention, response inhibition, and working memory while disengaging DMN nodes.
A key next step was to demonstrate that deactivations detected in DMN nodes were neural in origin. They could have been artifacts of baselines and thresholds used in PET and fMRI data analyses, or they may have reflected vascular or metabolic rather than neural changes. In a study involving three patients with intractable epilepsy, researchers recorded electrical potentials directly from the mPFC and the PCC, precuneus. They observed a selective increase in high-frequency gamma band power, 76 to 200 Hertz, during the resting state when compared with activity during finger movement, vision, and speech tasks. Transient neural suppression in the high gamma band has also been detected in the mPFC and PCC during spatial attention and word reading tasks and in the PCC and RSC during mental arithmetic. These studies provided replicable electrophysiological evidence for suppression of neural activity within the DMN core during a wide range of cognitive tasks. Thus, by 2011, intracranial EEG studies had firmly established a neuronal origin for DMN suppression during cognition. Subsequent studies have confirmed and extended these findings. Additionally, analysis of the directionality of information flow between the DMN and the salience and frontoparietal networks revealed causal outflow hubs in the salience network, consistent with its role in fast temporal switching of network interactions. Together, these findings identify a putative network mechanism by which the human DMN is suppressed during cognitively demanding tasks. More recently, these findings have been validated using optogenetic stimulation and fiber photometry in the rodent brain, Figure 4.
In sum, suppression of the DMN and network switching to engage the cognitive control networks are implemented by a common mechanism and involve flexible causal hubs anchored in the salience network. These task-induced dynamical processes contribute to the ubiquitous process of deactivation of the DMN and its disengagement from cognitive control systems widely engaged by tasks that require attention and working memory resources.
Direct role of the DMN in cognition.
Research over the past two decades has greatly expanded our understanding of the direct role of the DMN in human cognition. Meta-analyses of more than 8,000 task-based fMRI studies have provided strong evidence for a direct role of the DMN in human cognition, see Figures 5 and 6.
Figure 4. Suppression of DMN by optogenetic stimulation of anterior insula.
(A) Feedforward optogenetic stimulation of neurons of the right anterior insular (AI) during fMRI in Chronos-expressing rats. Extracted time-series data from ROIs corresponding to putative DMN and salience network nodes.
(B) Histological confirmation of EYFP viral vector expression (green) in the dorsal agranular insular cortex (AID), ventral agranular insular cortex (AIV), and dysgranular insular cortex (DI) subdivisions. Optical fiber placement in the AID and AIV subdivisions of the right AI (green).
(C) Right AI, cingulate (Cg), prelimbic cortex (PrL), and six subdivisions spanning the anterior-posterior axis of the RSC.
(D and E) AI stimulation resulted in activation of AI and PrL and suppression of multiple subdivisions of the RSC nodes of the rodent DMN.
(F) Functional connectivity decreased between the AI and an anterior subdivision of the RSC, within the RSC, and between the PrL and a mid-RSC subdivision.
I highlight five main areas of focused research, each of which has brought unique experimental methodologies and perspectives into our understanding of key cognitive functions subserved by the DMN, including self-reference, social cognition, episodic memory, and its role in personally relevant aspects of remembered experiences, language comprehension and semantic memory, and mind wandering. For the most part, investigations into these cognitive domains have proceeded independently. Here, I synthesize key findings, emphasizing the contributions of individual DMN nodes in relation to specific experimental manipulations. The insights gained shed light on the dynamic and overlapping roles of individual DMN nodes at the nexus of memory, language, and semantic operations.
Self-referential judgments.
A critical initial line of evidence for the DMN’s direct role in cognition emerged from studies on self-other judgments. These studies, which involved tasks related to self-traits and self-other distinctions, were among the first to demonstrate that DMN nodes could be activated above resting baseline. In a canonical version of such tasks, participants are presented with trait adjectives, such as timid, polite, and perfectionistic, and asked whether the words describe them.
Studies comparing self-referential and resting-state processes have identified overlapping activation in the mPFC, PCC, and left AG. Notably, all three areas exhibit enhanced activation during self-referential processing compared with resting baseline activity. The distinct roles of these DMN nodes in self-related judgments are not yet fully understood, but the mPFC appears to be particularly sensitive to differentiating self from close others.
Figure 5. Cognitive functions associated with the DMN contrasted with other brain networks Consistency of cognitive functions assessed using three different functional brain atlases.
The terms task-induced deactivations, theory of mind, semantic discrimination, episodic recall, emotion induction, and self-reflection figure prominently across atlases.
Proportion of voxels in the DMN, in relation to other large-scale networks in each map. ECN, frontoparietal executive control network, sal, salience network, precun, precuneus, BG, basal ganglia, visuospat, visuospatial network, sensmot, sensorimotor network, TIDs, task-induced deactivations. The ventral attention network by Yeo et al, includes the salience network. The language network by Shirer et al, includes large parts of the DMN as depicted by others.
Additionally, effective connectivity analysis suggests that the PCC may upregulate activity of other DMN nodes during self-related mental processes, consistent with its role as a hub node in the DMN. In contrast, the mPFC is more specifically engaged by demands for cognitive elaboration, evaluation, and emotion reappraisal, whereas the left AG is involved in the retrieval of semantic and personally relevant information.
In summary, the mPFC, PCC, and left AG constitute a core self-referential system within the DMN, sustained by activity in the PCC and regulated by feedback from the mPFC whose influence is enhanced with more effortful self-directed cognition.
Social cognition.
Self-referential mental processes facilitate reasoning about oneself in the context of social interactions with others. Consistent with this perspective, the DMN is implicated in a broad range of tasks probing social cognition and communication, with consistent activation patterns reported in the PCC, mPFC, AG, and adjoining temporal parietal junction. DMN activity also predicts the trajectory of current and future mental states of others. Dissociable roles for the PCC and mPFC in social functioning have been reported, with the PCC differentially involved in self-other distinctions and the mPFC in monitoring both one’s own and others’ mental states. The right AG and adjoining temporal parietal junction preferentially encode other relevant information, such as the mental states and beliefs of others, and predict others’ behaviors during social interaction.
An innovative research direction using naturalistic stimuli has demonstrated that ongoing DMN activity of two individuals becomes synchronized when they process shared narratives, with DMN synchronization being particularly sensitive to social communicative cues. Recent studies have also shown that inter-individual neural synchronization in the DMN is related to social proximity, reflecting evaluation of whether other individuals are friends, friends-of-friends, or more distally connected in social ties. Inter-individual DMN synchronization may underlie similar mental representations, reflecting shared values and beliefs, as well as a similar style of perceiving the world.
In summary, the DMN consistently responds to various aspects of social content and context, encompassing the consideration of mental states, making inferences about others’ mental states, and establishing shared meaning and narratives about the self in the world.
Episodic memory.
The DMN plays a crucial role in episodic memory operations, particularly those related to personally relevant details of remembered experiences. Early evidence for the DMN’s role in episodic memory emerged from studies in patients with Alzheimer’s disease. Significant deficits in coactivation of the hippocampus with other DMN nodes suggested that this network is closely involved in episodic memory processing. Building on these early findings, a vast body of research has demonstrated the consistent and replicable engagement of individual DMN nodes in memory encoding, retrieval, and free recall. The DMN’s role in autobiographical memory has been well articulated in previous reviews.
Episodic memory relies on interactions between the hippocampus and its interconnected cortical circuits, with the PCC, RSC, mPFC, and left AG nodes of the DMN featuring prominently. These cortical nodes appear to be associated with different aspects of episodic memory: the PCC with autobiographical recall, RSC with spatial navigation, mPFC with regulation of memory encoding and recall, and the AG with elaboration of the contents of memory. The contributions of the MTL and cortical nodes of the DMN to memory operations can be dissociated, with hippocampal activity tracking successful encoding and retrieval and cortical nodes tracking recall of the detailed value and emotion-related features of autobiographical memory. DMN nodes also are crucial for conceptual knowledge of self and other-related schemas that support autobiographical memory.
The DMN has emerged as a central construct for investigating episodic memory and specifically the subjective experience of remembering. Recent reviews have proposed that subnetworks associated with the DMN underlie episodic memory construction and relational binding within an egocentric perspective. A DMN-based framework suggests that no single brain region is solely responsible for the subjective experience of episodic memory. Rather, the constituents of episodic memory are influenced and transformed by multiple representations engaging distributed DMN nodes.
Language and semantic memory.
DMN nodes in the lateral temporal and lateral parietal cortices are consistently implicated in language comprehension and semantic processing. Overlap with the speech and language processing system is particularly evident in the left AG, anterior temporal cortex (ATC), and middle temporal gyrus (MTG) across a wide range of semantic tasks that assess the ability to use, manipulate, and generalize knowledge. Specific associations of DMN nodes at the interface of language and semantic cognition include AG with semantic judgments, MTG and adjoining superior temporal sulcus with language and speech comprehension, and ATC with semantic memory and categorization.
Meta-analyses have confirmed significant overlap between the DMN and language and semantic processing systems. A key DMN node at the intersection of these systems is the PGp subdivision of the AG. Area PGp is involved not only in semantic categorization of individual words but also in speech intelligibility and sentence comprehension.
Figure 6. Probabilistic analysis of cognitive functions associated with task-related activation and inter-regional coactivation of four key DMN nodes:
(A) Cognitive functions associated with activation of mPFC, PCC, and left and right AG nodes of the DMN, across 14,000 task-related studies. Log-odds ratio (LOR) of the cognitive term being reported:
Logarithm of p (term reported in a study, a focus within 4 millimeters of the DMN node reported in the millimeters study) over p (term reported in a study, no focus has been reported within 4 millimeters of the DMN node in the study.
(B) Cognitive functions associated with coactivation of mPFC, PCC, and left and right AG nodes of the DMN taken pairwise. Data generated using NeuroLang.
(C) Meta-analysis of task-related coactivation patterns of the mPFC, PCC, and AG. The PCC and mPFC show strong coactivation. Only the mPFC is coactivated with amygdala and ventral striatum, left AG is coactivated with left MTG, and the PCC is differentially coactivated with hippocampus. Hipp, hippocampus, Amyg, amygdala, VS, ventral striatum.
A recent analysis of five different studies revealed that area PGp is one of the few brain regions that shows activation above the resting-baseline during tasks involving semantic judgment.
Research using naturalistic speech stimuli has provided valuable new insights into DMN involvement in language-based communication. Speech unfolds over time, and comprehension of extended verbal conversations requires active prediction as listeners anticipate incoming speech as a means of inferring content and meaning. Employing a novel experimental paradigm that modeled the listener’s brain activity from a speaker’s activity, it was found that speaker and listener brain activities were temporally correlated in the mPFC and PCC nodes of the DMN. Additionally, brain responses of the listener in the mPFC predicted brain activity of the speaker while responses in the PCC reflected reaction to the speaker’s brain activity, and the strength of this prediction was correlated with narrative comprehension.
In summary, the DMN plays a role in the semantic and narrative aspects of speech processing, as well as the predictive and anticipatory aspects of language comprehension. Moreover, research is emphasizing the complex interplay between language and semantic memory while revealing the engagement of the DMN in both external and internal speech.
Mind wandering.
Mind wandering refers to thought processes that are unrelated to current executive task goals. These shifts in attention from stimulus-based processing to introspective cognition are ubiquitous in everyday life. Investigations into spontaneous internal thoughts associated with mind wandering have played a pivotal role in uncovering the broader function of the DMN in human cognition.
An early groundbreaking study used in-task thought-sampling probes to obtain dynamic, real-time measurements of mind wandering during cognition. Participants were intermittently asked to self-report their current mental states. Activation of the mPFC, precuneus, and bilateral AG was detected during the time interval immediately preceding mind wandering. Furthermore, activation in the mPFC was observed in connection with both subjective self-reports of mind wandering and performance errors in a dual target detection task. Meta-analysis of 24 functional neuroimaging studies of spontaneous thought processes identified consistent recruitment of the mPFC, PCC, MTL, and AG.
Further support has been provided by studies in patients with brain lesions, which revealed a strong association between reduced mind wandering and left AG lesions. Meta-analyses of neuroimaging studies exploring episodic future thinking, mind wandering, and personal goal processing have found that these three domains activate a shared set of regions within the DMN, with the mPFC being particularly prominent. Findings using multiple methodologies have provided replicable evidence for the DMN’s involvement in mind wandering and spontaneous stimulus-independent thoughts. Lesion studies suggest a causal role for the DMN in this process.
Unifying themes and perspectives on DMN function.
Here, I describe a unifying model that synthesizes key themes that have emerged in the literature on the DMN over the past 20 years, Figure 7. The model emphasizes the importance of understanding the dynamic interactions between the DMN and other brain networks to gain a comprehensive understanding of the DMN’s role in cognitive processes. As described above, individual nodes of the DMN have been implicated in a wide range of cognitive functions, including self-reference, social cognition, episodic memory, and language and semantic memory. I propose that the DMN integrates these cognitive functions to create a coherent internal narrative of our experiences. The model emphasizes the DMN’s role as a functional and structural hub in the brain, assimilating and transmitting representations of salient external and internal events through global brain activity patterns. These patterns are intermittently altered by latent switching mechanisms, leading to new brain states and mind wandering.
Salient stimulus-driven cross-network interactions shape DMN role in cognition.
The role of the DMN in cognition was initially believed to be limited to encoding internal thoughts that needed to be suspended during external stimulus processing. This idea was supported by evidence of reduced DMN deactivation leading to behavioral errors during attention-demanding tasks. Studies using multiple experimental paradigms, recording techniques, and computational modeling have advanced our understanding of the underlying neural mechanisms, and these suggest that the DMN’s indirect contribution to cognition arises from failures of disengagement due to weak network switching. Notably, suppression of the DMN and engagement of cognitive control networks during task performance are thought to be controlled by a common mechanism, with the salience network playing a crucial role.
The triple-network model posits that the salience network plays a key role in processing behaviorally relevant external events, leading to suppression of the DMN and altered temporal interactions between the DMN, salience, and frontoparietal networks, Figures 3 and 7. These dynamic processes result in disengagement of the DMN from cognitive control systems, enabling focused attention and working memory for goal directed behaviors. In this framing, salient stimuli induce external event-driven network switching that shapes the dynamic role of the DMN in cognition. Thus, the functional role of the DMN must be considered within the context of its interactions with other cognitive control networks, which enable task and context-specific network switching.
More generally, by virtue of their unique connectivity and co-activation patterns, individual DMN nodes may contribute to distinct aspects of network function. Based on the extensive research reviewed above, it would be erroneous to conceptualize the DMN as a static network with a unitary function. Moreover, the functional dynamics of the DMN are far from stationary, and multiplexed subnetworks associated with individual DMN nodes provide functional streams by which the DMN can dynamically couple with other networks. A common theme that emerges here is that the DMN does not operate in isolation, and its functions are shaped by dynamic interactions with other brain systems.
DMN nodes are involved in multiple overlapping cognitive functions.
The principal basis for interpreting the direct role of the DMN in cognition has been a node level view. Although not integrative, this view is based on an extensive body of empirical research demonstrating the involvement of its constituent nodes in various cognitive domains. The cognitive processes reviewed above are not mutually exclusive, and there are multiple points of intersection in terms of the underlying neurocognitive processes, a view reinforced by meta-analytic investigations.
Several meta-analyses have confirmed the involvement of the DMN in multiple cognitive functions and identified their distinctive properties as well as points of convergence with other large-scale networks, Figures 5 and 6. Importantly, DMN nodes are not uniformly engaged across cognitive domains, and several distinct features have emerged. For example, the PCC and mPFC are differentially involved in self-other distinctions, RSC in spatial memory, precuneus in visual imagery, the hippocampus in episodic memory formation, the ATC in semantic memory, the left AG in language-related semantic judgments, the right AG in social-evaluative functions, the left MTG in monitoring internal speech, and the mPFC in value judgments, emotion regulation, and generation and elaboration of stimulus-independent thoughts. These distinctions further reinforce the view that the DMN does not serve a unitary function, at least not in the way cognitive functions are currently conceived, labeled, and compartmentalized.
Figure 7. Unifying model of DMN role in cognition.
The model posits four key unifying themes that underlie DMN function:
(1) Network switching shapes DMN role in cognition. Salient stimuli result in suppression of the DMN during attention demanding tasks. This suppression is followed by a rebound and return to internally focused mental processes. Multiple lines of evidence suggest that the anterior insula plays an important role in suppression and disengagement of the DMN.
(2) DMN nodes link multiple cognitive functions associated with self-referential judgments, social cognition, language and semantic memory, and episodic memory operations.
(3) The DMN integrates these cognitive operations to construct an internal narrative.
(4) The DMN sustains frames of thought, and its hub properties facilitate network switching and global state changes. This dynamic process creates new frames of thought. Mind wandering, one of the cognitive functions ascribed to the DMN, is a natural outcome of this dynamical process.
In summary, going beyond individual studies, meta-analytic findings confirm that individual DMN nodes and their connections are involved in multiple overlapping cognitive functions. Their involvement in both internally directed and external stimulus-related cognitive processes calls into question a strict dichotomy and rigid distinction between the two and supports the notion of an integrative role for the DMN in cognition.
An integrative system for sustaining inner narratives.
How do the various functions ascribed to the DMN come together to serve a larger overall function? I propose that the DMN is an integrative system that facilitates the construction of an ongoing internal narrative drawing on the key cognitive elements I have highlighted above. These narratives shape our understanding of our individual, highly personal, experiences. Here, it is well to ponder a gedanken experiment on what human cognition might look like if the DMN stopped functioning. I suggest that this would effectively end the narratives we tell ourselves. Our brains would not bind experiences to create a coherent internal narrative in the context of our experiences and shared social interactions. This narrative, part monolog and part dialog, is central to construction of the ‘‘epistemic self,’’ component processes of which include episodic memories, semantic knowledge of facts about one’s life and the world, representations of individual values and beliefs, and the ability to experience and produce evaluative directions to our perceptions, actions, and reasoning.
Such narratives necessarily involve integration of operations subserved by language, semantic, and memory systems that intersect in the DMN.
I further posit that the DMN integrates component processes and broadcasts them globally to construct and maintain an experience of subjective continuity. This integrate-and-broadcast process is briefly paused when stimuli in the external world need to be attended to. Once they are attended to, the inner narrative resumes with updated representations of events that are personally salient. This process allows us to build mental models of the world and integrate new information for further deliberation and reflection, constrained by our past experiences but removed from the exigencies of external stimuli and task contexts. Disruptions to any stage of this process can render the system vulnerable to a loss of sense of self, as seen most dramatically in psychiatric conditions such as dissociative disorders and neurological disorders such as Alzheimer’s disease.
I speculate that the DMN’s role in constructing such an inner narrative may have ontogenetic origins in early childhood. In his seminal work on thought and language, Vygotsky proposed that inner speech is the internalization of external verbal communication, a process by which we take in and internalize the words and language that we hear from others and then use this internalized language, now appropriated for the self, to think, reflect, and communicate with ourselves and others. Vygotsky articulated a developmental basis for this process in early childhood. He argued that children initially use self-directed speech to regulate their own actions and thoughts. Over time, children and adults internalize this self-directed private speech into a form of abstract voiceless inner monolog, which then becomes an efficient tool for self-regulation, planning, and social communication.
Broadcasting frames of thought.
DMN nodes are well placed to integrate and broadcast neural representations. Several lines of research have highlighted the unique structural and functional characteristics that enable DMN nodes to serve as a signaling hub in the brain, Figure 8. The hub-like organization, characterized by a short path length and local and global hubs in the PCC, may enable the DMN to connect and influence other networks, thereby facilitating global state changes. DMN nodes are located at one extreme of a functional hierarchy, along a spectrum from unimodal to heteromodal brain regions, allowing it to integrate structured information from multiple brain areas. Additionally, dynamic causal modeling applied to human fMRI and intracranial EEG data point to a sustained causal influence of the DMN on other brain networks. Surprisingly, even in the resting state, the PCC and mPFC nodes of the DMN have been shown to exert greater causal influences on lateral frontoparietal networks than the reverse. Building on this finding, information theoretic analysis of intracranial EEG signaling across widely distributed depth electrodes revealed that the DMN had significantly greater net causal outflow to other brain networks including sensory and motor networks than the reverse, during the resting state and during episodic memory formation, and the strength of causal interactions was higher during memory formation compared with the resting state.
These global spatiotemporal dynamical properties may support frames of thought, in which the DMN plays a key role in integrating and transmitting representations of salient sensory and internal events in spatial patterns of brain activity that resemble frames in a movie. In this view, integration, along with transmission of neural representations by the DMN, is a dynamic process that occurs in distinct stages, including the accumulation of information over time, the identification of event boundaries, and the alteration of network configurations that result in global state changes. There is growing evidence that the DMN integrates information over slower timescales than other brain systems, as demonstrated in language-based narrative (speech) processing. Neural activation patterns in the PCC tend to persist for extended periods of time during naturalistic movie watching, and transition points coincide with perceived event boundaries. The DMN has also been shown to represent event boundaries in both external narratives and internally generated boundaries of mental context during unguided narrative recall, with neural patterns that generalize across both internally and externally driven boundaries being most strongly observed in the PCC and RSC.
The mechanisms by which neural representations may be transmitted and integrated to create new global states in the human brain are not yet understood. However, recent studies in rodents have provided insights into how this process may occur. A two-photon calcium imaging study in rodents found that different areas of the brain shared task-related information and connectivity within 200 milli-seconds of a sensory stimulus and that a global fluctuation mode conveyed the animal’s response to every brain area examined within 1 second of stimulus presentation. This process appears to communicate information in modes orthogonal to those carrying sensory data, allowing for the global broadcast of current behavioral states without loss of information about external stimuli. A similar process may also support the global broadcast of brain states and frames of thought in the human brain.
To summarize, I have articulated a model of DMN function based on four key unifying themes that have emerged in the literature, Figure 7. First, salient stimuli induce external event-driven network switching that shapes the dynamic role of the DMN in cognition. Processing of salient stimuli results in suppression of the DMN during attentionally demanding tasks, and this suppression is followed by a rebound and return to internally focused mental states. Second, DMN nodes are involved in multiple overlapping cognitive functions as determined with experimental paradigms that manipulate various aspects of self-reference, social cognition, language and semantic memory, and episodic memory. Third, the DMN integrates cognitive operations supported by its individual nodes to sustain an internal narrative characterized by distinct brain states and frames of thought. Fourth, the hub properties of the DMN facilitate internal event-driven network switching and global state changes, which lead to new frames of thought. Mind wandering, one of the cognitive functions ascribed to the DMN, is a natural outcome of this dynamical process. Appropriately directed, these frames of thought and shifts in frames of thought (mind wandering) may support creative problem-solving, while misdirection of this process leads to attentional lapses and maladaptive behaviors.
Figure 8. Global hub and spatiotemporal properties of the DMN.
(A) Functional connectivity density maps showing PCC, precuneus are both local and global hubs in the brain. Adapted from Tomasi and Volkow.
(B) Graph theoretical analysis of structural connectivity reveals high degree and betweenness centrality of the mPFC and PCC-RSC, as well as the basal forebrain and thalamus. The size of each node represents its degree, and the node color illustrates node betweenness centrality. Adapted from Alves et al.
(C) The DMN is located at the apex of gradients that place it functionally and spatially distant from primary sensory and motor networks. Adapted from Smallwood et al.
(D) Causal network influences of the DMN on six other large-scale brain networks examined using brain-wide intracranial electrophysiological recordings. Phase transfer entropy (PTE) reveals higher net causal outflow from the DMN, than the reverse, during episodic memory formation.
(E) Causal influences of the DMN on other brain networks is higher during both episodic memory encoding and recall, compared with resting state.
(F) DMN displays higher net causal outflow to other brain networks (DMN, other) than the reverse other, DMN. Adapted from Sridharan et al.
Emerging perspectives on DMN anatomy, subnetworks, and multiplexed circuits.
Our understanding of DMN anatomy and physiology has evolved significantly in recent years. The prevailing view has been that the DMN functions as a singular system anchored in the PCC. However, recent studies have revealed significant levels of heterogeneity and segregation of the DMN into interdigitated subnetworks. Within the posterior medial parietal cortex, the dorsal PCC, ventral PCC, and RSC may anchor subnetworks associated with distinct roles in cognitive control, mnemonic, and spatial processes. The application of high-resolution individualized approaches suggests that PCC subdivisions are differentially activated by distinct aspects of internal mental representations. Additionally, there is growing evidence that the dorsal and ventral PCC interact differently with other DMN and cognitive control networks. Task-based analyses also suggest a fractionation of cognitive processes across subnetworks.
These findings challenge earlier notions of an undifferentiated PCC as a global hub and the DMN as a network with a unitary function. One way to reconcile these findings, within the context of the integrative model proposed here, is that the DMN comprises distinct subnetworks that subserve dedicated cognitive functions. However, these subnetworks are not completely segregated and likely contain convergence zones resulting in interdigitated and multiplexed circuits. These convergence zones may serve as connector hubs that integrate information across DMN subnetworks, thereby facilitating flexible and adaptive integration of self-referential, social, episodic memory, language, and semantic memory processes described in this review.
Challenges and future directions.
What is the function of the DMN? The answers to this question have been remarkably varied, and there is still much we do not understand. While considerable progress has been made in probing the functional organization of the DMN and its indirect and direct roles in external stimulus-related cognition, several fundamental questions remain. In addressing these challenges, we are presented with a conundrum similar to one faced by physicists a century ago: internal mental processes cannot be studied without external stimuli, yet once exposed to external probes, internal mental and brain states are altered and disrupted, similar to the Schrodinger effect in physics. Improved experimental perspectives, neural recording methodologies, and computational models are required to address the many remaining open questions.
Many crucial aspects of global brain dynamics needed to uncover the dynamical properties of the human DMN are beyond the temporal resolution of current brain imaging techniques. The use of EEG and MEG to characterize network dynamics remains a challenge due to limited spatial resolution, which is further confounded in the case of DMN nodes PCC, RSC, and mPFC, which are buried in the medial cortical surface, and the hippocampus that is further deep in the MTL. Intracranial EEG recordings offer a potential avenue for addressing this challenge, but the limited number of participants and the lack of dense electrode coverage across distributed nodes that comprise the DMN have made it difficult to probe its neurophysiological properties and its functional network dynamics during cognition. A particular challenge is obtaining intracranial EEG data from DMN nodes across multiple lobes in the same individual. Moreover, core DMN nodes in the PCC, RSC, and ventral mPFC are not typical seizure foci in patients undergoing surgery for intractable epilepsy.
The lack of temporal precision in fMRI has made it challenging to trace the flow of signals and representations from the processing of external stimuli to the suppression or activation of DMN and the subsequent global signaling that I have hypothesized. A further challenge is to determine how global hierarchical brain organization, characterized by DMN nodes exhibiting the greatest geodesic distance from primary sensory and motor cortices, as well as association cortices, influences cognition. This leaves it unresolved whether destabilization of brain states occurs because of external stimuli or because of internal processes that in turn may have been triggered by external stimuli. A further challenge is to elucidate how and under what conditions the ensuing global brain dynamics impacts ongoing internal mental processes.
Recent studies have provided evidence that the DMN is not a singular, homogeneous system, instead, it comprises distinct subnetworks that perform different cognitive functions. The precise functional anatomy of subnetworks, the convergence zones that link them, and their distinct contributions to cognitive functions remain an area of active research. It is likely that some convergence zones reflect the processing of internally generated information, such as autobiographical memories or future-oriented thoughts, while others are involved in the integration of information across multiple sensory modalities. The heterogeneity of the DMN also poses a challenge in understanding its interplay between deactivation and activation. These subnetworks may have distinct patterns of activation and deactivation during different cognitive processes, which could contribute to the complexity of understanding the interplay between deactivation and activation in the DMN as a whole. Further research is needed to better understand the nature of these subnetworks and convergence zones, individual variability in DMN organization, and their relation to cognitive function and behavior.
The inherent constraints of current human imaging methodologies limit our ability to probe cellular mechanisms underlying the operations of the DMN. Translational studies in rodents offer possibilities for addressing critical gaps in our knowledge of network function and developing a deeper understanding of the mechanisms underlying DMN functions. The advent of multiplexed genetically encoded calcium sensor recordings and optogenetics has made it possible to record and manipulate cell-type-specific ground-truth neuronal activity in rodents, studies that are currently implausible in humans. Crucially, causal manipulation of specific DMN nodes with optogenetic tools offers the possibility of significantly enhancing our understanding of the foundational cellular and neural-circuit bases of the DMN and its dynamic brain network organization.
The translational utility of these models is currently limited by an incomplete understanding of rodent DMN physiology and function, but progress is being made. At the same time, it should be noted that the many unique aspects of human DMN function, including mind wandering, language, and semantic cognition, will likely remain outside the purview of rodent and even non-human primate investigations. Finally, developmental perspectives on DMN function have been largely missing as most research in children and adolescents to date has tended to focus on resting-state connectivity. Task-based fMRI studies, using relevant self-referential, language, and memory paradigms, as described above, will be needed to determine how cognitive functions of the DMN and its role in internally focused mental life emerge and develop through childhood and adolescence. Longitudinal studies are needed to clarify how narrative identity and meaning making arise from internalization of self-directed speech and how social interactions contribute to beliefs, personality growth, and the emergence of a sense of the self.
Conclusions.
The discovery of the DMN and two decades of subsequent work have deepened our understanding of how the human brain produces complex behaviors, including our inner mental lives. I have reviewed events and milestones in the study of the DMN, beginning with its discovery, and highlighted advances from theoretical and experimental perspectives. I have sought to reflect on the discovery process, in the hope that it may be useful for making the next generation of advances in the field. I have described the indirect and direct roles of the DMN in human cognition, which are interrelated. The indirect role arises from inefficient and inappropriate suppression of internal mental processes. The direct role encompasses cognitive operations associated with self-reference, social cognition, episodic and autobiographical memory, and language and semantic memory. Research in each of these areas has brought unique experimental methodologies and advanced our understanding of the cognitive functions of the DMN.
The unifying model I have outlined posits that the DMN combines episodic memory, language, and semantic memory processes to generate an ongoing internal narrative and subjective continuity of internal mental thoughts. This narrative, which is unique to each of us, plays a critical role in the construction of a sense of self, encompassing the individual self and the social self. The individual self embodies our ability to introspect and to be cognizant of our thoughts, emotions, and actions. The social self involves perspective taking, navigating social situations, forming and maintaining relationships, and understanding one’s behaviors within a social context. Together, they forge a multifaceted personal narrative that shapes how we perceive ourselves and
-
17:17
DeVory Darkins
18 hours agoKamala Post-Election BOMBSHELL Exposes $1 BILLION Campaign DISASTER
19.6K107 -
19:52
Stephen Gardner
23 hours ago🔥HOLY CRAP! Trump just did the UNTHINKABLE!!
35K379 -
4:34:55
Pepkilla
6 hours agoBlackops Terminus Zombies Boat Glitch
97.9K4 -
5:50
CapEx
18 hours ago $22.59 earnedWhat the Coming & Inevitable Sovereign Debt Crisis Means for YOU | CapEx Insider
96.8K25 -
1:34:00
Tactical Advisor
7 hours agoAR15 Giveaway WINNER/Trump Winning | Vault Room Live Stream 008
64.2K25 -
5:41:10
Vigilant News Network
8 hours agoOfficials CAUGHT Changing Ballots in Arizona | The Daily Dose
113K42 -
17:30
Forrest Galante
1 day ago5 Most Dangerous Invasive Species in the World
84.7K52 -
38:53
Popcorn In Bed
1 day agoTOP GUN: MAVERICK | FIRST TIME WATCHING | MOVIE REACTION
98.6K21 -
0:56
scoutthedoggie
1 day agoAirsoft Tracer Wars
91.6K19 -
10:23
Space Ice
1 day agoSteven Seagal's Urban Warfare Is A Real Movie, I Promise - Worst Movie Ever
68.4K33