Premium Only Content
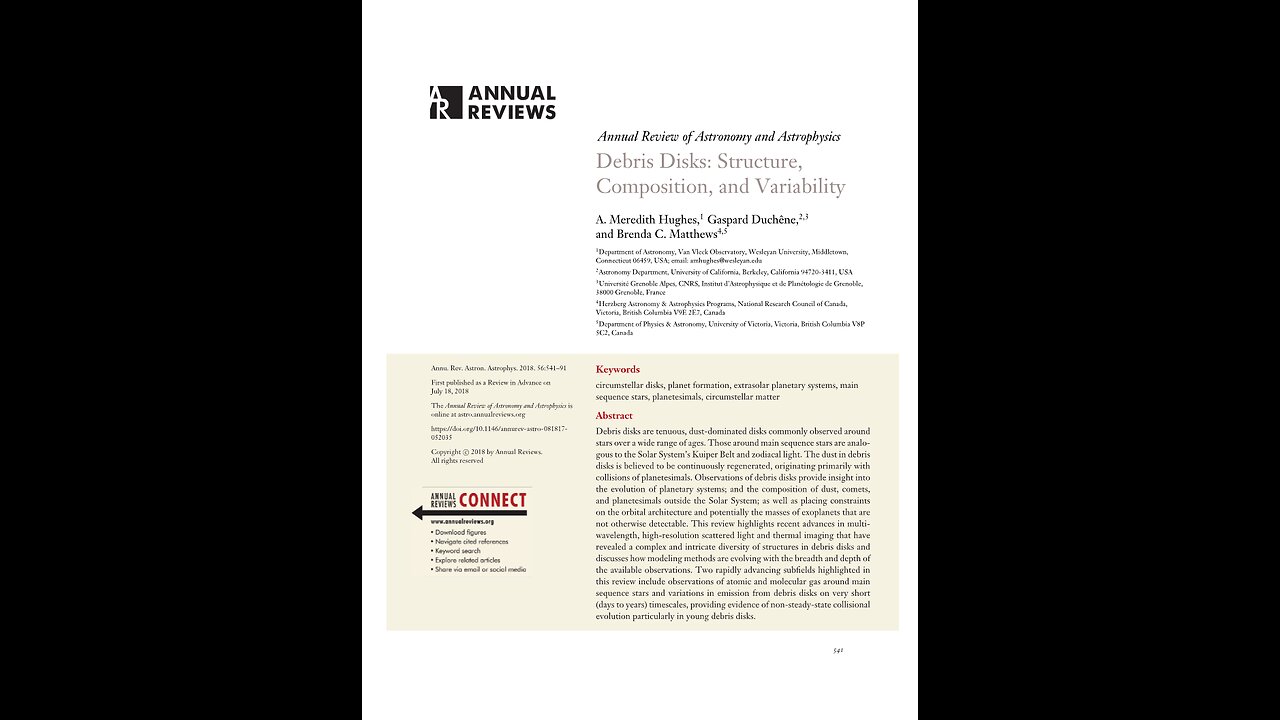
Debris Disks: Structure, Composition, and Variability. A Puke(TM) Audiopaper.
Annual Review of Astronomy and Astrophysics.
Review Article.
Debris Disks: Structure, Composition, and Variability.
Annu. Rev. Astron. Astrophys. 2018. 56:541–91
https://doi.org/10.1146/annurev-astro-081817-052035
A. Meredith Hughes, and others.
email: amhughes@wesleyan.edu
Index of Other Science Articles:
https://rumble.com/v3t4yzj-index-of-science.-music-by-dan-vasc.html
Debris Disks: Structure, Composition, and Variability.
Meredith Hughes, and others.
Keywords:
Circumstellar disks, planet formation, extrasolar planetary systems, main sequence stars, planetesimals, circumstellar matter.
Abstract.
Debris disks are tenuous, dust-dominated disks commonly observed around stars over a wide range of ages. Those around main sequence stars are analogous to the Solar System’s Kuiper Belt and zodiacal light. The dust in debris disks is believed to be continuously regenerated, originating primarily with collisions of planetesimals. Observations of debris disks provide insight into the evolution of planetary systems, and the composition of dust, comets, and planetesimals outside the Solar System, as well as placing constraints on the orbital architecture and potentially the masses of exoplanets that are not otherwise detectable. This review highlights recent advances in multi-wavelength, high-resolution scattered light and thermal imaging that have revealed a complex and intricate diversity of structures in debris disks and discusses how modeling methods are evolving with the breadth and depth of the available observations. Two rapidly advancing subfields highlighted in this review include observations of atomic and molecular gas around main sequence stars and variations in emission from debris disks on very short (days to years) timescales, providing evidence of non-steady-state collisional evolution particularly in young debris disks.
One. Introduction.
1 point 1. What Is a Debris Disk?
Debris disks are both an outcome and an integral component of the formation of planetary systems. From the earliest stages of star formation to the final planetary systems, circumstellar disks of material are present, but these disks change their structure, dynamics, and composition significantly over time. In the earliest phases of star formation, a pre-main sequence star forms surrounded by a gas-rich protoplanetary (or planet-forming) disk of gas and dust. Transition disks refer to a phase between a protoplanetary disk and a debris disk, but in this epoch, disks are still characterized by high gas-to-dust ratios and (often) continued gaseous accretion on the central star. Eventually the transition disk dissipates through some combination of stellar and planetary mechanisms involving accretion, photoevaporation, winds, and agglomeration of large solid bodies. The timescale for the decrease in emission from such disks is wavelength dependent, with most disk emission gone by 3 Mega years in the near-IR (NIR) and 20 Mega years in the submillimeter.
Collisional cascade.
The term collisional cascade refers to the process by which planetesimals are gradually ground to submicron-sized dust grains. The collision of the most massive bodies ignites the cascade by producing many smaller objects that then collide with one another to create even more smaller bodies, and so on until the smallest bodies are the most numerous even while the bulk of the mass is retained in the largest bodies. For a self-similar, steady-state cascade, the differential size distribution within the cascade follows a power law such that dN, da is proportional to a to the minus q, where a is the grain size and q is around 3.5. Numerical analyses support this general behavior, albeit with departures from a pure power law.
Debris disks, known also in the literature as secondary disks, are not leftover remnants of the protoplanetary or transition disks, but instead they must be continuously sustained by collisional processes, the steady-state evolution of disks is typically modeled as a collisional cascade. See the sidebar titled Collisional Cascade, to be observable over the lifetime of the star. Radiation forces, stellar winds, and Poynting-Robertson drag forces all act to continuously clear dust grains smaller than the blowout size from orbits around the star, see the sidebar titled Blowout Size. Thus, the detection of a debris disk indicates that planet-formation processes in the system were successful in forming bodies of at least several hundreds to thousands of kilometers in size. Collisional cascades grind the material down to small dust grains observable in both scattered light and thermal emission.
Protoplanetary disks and debris disks represent distinct classes of objects. Physically, proto planetary disks can be regarded as agglomeration dominated, whereas debris disks are dominated by destructive processes. Both types of disks can be detected through excess emission relative to the stellar photosphere at wavelengths that can range from the NIR to the submillimeter. Unlike protoplanetary disks, however, for which evolution of the disks is mirrored by predictable changes in the degree and wavelengths of excess, debris disks may lack excess emission at some wavelengths simply because the disks are not radially continuous or because of the distribution of mass across the disks. Debris disk emission may also vary significantly owing to perturbation of the disk material that renders them brighter or fainter, sometimes over very short timescales.
Blowout size.
The blowout size is the largest grain size in a disk that can be expelled directly by stellar pressure forces. For grains at the blowout size or smaller, the radiation pressure of a solar or earlier-type star effectively transports the grains out of the disk on hyperbolic orbits, forming the halo, see Figure 1. The luminosity of low-mass stars is too low to effectively expel grains of any size. However, their strong stellar wind can also act to remove the smallest dust grains through ram pressure, thus defining a blowout size. Generally speaking, the blowout size is defined as the grain size for which the force ratio:
Beta forces equals F radiation plus F wind over F gravity is greater than or equal to a half, where F rad, F wind, and F grav are radiative pressure, wind ram pressure, and gravity forces, respectively. The blowout size sets a lower bound to the size distribution produced by the collisional cascade in the disk. The minimum grain size derived from observations is often larger than the blowout size, likely as a result of a change in the microphysics of grain collisions in the small grain limit.
Nonetheless, the presence of excess thermal emission is generally the most effective diagnostic of the presence of circumstellar dust. However, distinguishing observationally between the two classes is nontrivial. Various criteria have been proposed in the literature, but nearly all rules either have clear exceptions or rely heavily on assumptions about poorly constrained properties of the system. A system’s age is not a good criterion because stellar clusters with ages of around 5 to 15 Mega year contain coexisting examples of both protoplanetary and debris disks, and even clusters as young as 2 to 3 Mega year show signs of incipient debris disks. The absence of molecular gas was historically a distinguishing characteristic of debris disks, but evidence of gas is now detected toward many debris disks (see Section 4). The most practical criterion seems to be optical depth: The dust in debris disks is optically thin across the electromagnetic spectrum, whereas the dust in protoplanetary disks is typically extremely optically thick at optical wavelengths and may remain optically thick in the inner regions even into the millimeter part of the spectrum. The observational proxy now used most frequently to quantify the low optical depth of debris disks is the integrated fractional luminosity, f equals L disk over L star, also often presented as Tau equals L Infra Red over L bol. In practice, f is preferred as the more physical value, but Tau is more easily calculated for sparsely sampled spectral energy distributions, or S-E-D’s. In this review, we adopt an upper bound of Tau less than 8 times ten to the minus three to define a debris disk, selected somewhat arbitrarily to place HD 141569 right on this boundary, because it is the best candidate for a truly intermediate object between the protoplanetary and debris disk phase.
Figure 1.
Schematic of the potential components of a debris disk and an illustrative S-E-D. Depending on the system architecture, multiple planetesimal belts may be present, broad analogs to the asteroid and Kuiper Belts of the Solar System are shown here. Planets are shown as a means of confining dust into separate belts rather than to suggest any typical architecture. Through a steady-state collisional cascade, dust is generated in the planetesimal belts down to the blowout size. Stellar radiation and, or wind can displace, or completely remove, the smallest dust grains, populating analogs of the Solar System zodiacal light and of an extended outer halo that contains small dust grains on hyperbolic orbits and is best seen in scattered light images. The black arrows indicate the equilibrium 150 K blackbody distance from the star for three spectral types: M0V, G2V, and A0V. The positions of Earth and Neptune are shown for a sense of scale for this particular disk, the radial locations and radial extent of disk components can vary dramatically from one system to another. The illustrative S-E-D is for a model that contains each of these components, liberally adapted from the known structure of the Vega and Beta Pictoris debris disks. The system is built around an A0V star at 7.7 parsec, 237 peta-meters, and with a total integrated luminosity of Tau equals one point four times ten to the minus three. Dust temperatures in the model range from 30 K in the outer halo to as much as 2,000 K at the inner edge of the exozodiacal disk. Abbreviation: S-E-D, spectral energy distribution.
Our Solar System contains two components in its debris disk: the zodiacal light, which is composed primarily of material from disintegrations of Jupiter-family comets in the inner Solar System and the Edgeworth-Kuiper Belt, also known as the EKB or Kuiper Belt, consisting of material from collisions of comets between Neptune’s orbit and an outer bound of approximately 50 Astronomical Units, 7.5 Terra meters. Each of these components has Tau around ten to the minus seven. Disks detected around other stars are thus typically referred to as Kuiper Belt analogs (typically T less than 100 K) or exozodiacal dust belts, “exozodis” for short (typically T greater than 150 K). Extrasolar debris disks often show evidence of multiple components, reinforcing the idea that planets are common in debris systems, because planets are a primary, but not the only, means of excluding dust from certain regions of a disk.
Figure 1 shows a schematic of a debris disk’s components. Generally, the components associated with a terrestrial planet zone are referred to as the inner disk, characterized by warm or hot dust emission and faster collisional evolution timescales. By contrast, the outer disk is characterized by cold dust in a Kuiper Belt analog in which collisional evolution timescales are longer. A radially extended halo of small grains may also be present at large radial separations from the host star. The relative distances of the 150-K blackbody equilibrium temperature for a range of stellar spectral types is also shown on Figure 1, illustrating how much more compact the terrestrial zone is for late versus early-type stars. Modern studies of planet formation strive to connect the initial conditions in a protoplanetary disk (gas and dust surface density, temperature, and velocity) to the final outcomes (exoplanet statistics) via models of planetary system formation and evolution. Historically, astronomers have tended to assume that protoplanetary disks provide information about the initial conditions, debris disks tell us about orbital evolution during the clean-up phase at the end of oligarchic growth, and exoplanets tell us about final configurations. The picture has become more complex recently, with mounting evidence that planets generally form quite early in the protoplanetary disk phase and that gas and dust in these systems are already significantly evolved relative to interstellar medium, I-S-M.
However, it is still clear that some phases of evolution must take place during the debris disk phase. Debris disks also provide a unique opportunity to study directly imaged planets and disks in the same system, which is much more difficult in protoplanetary disks due to their high optical depths and increased distances to their host stars. Due to their relative faintness, most debris disks currently studied lie within a few hundred parsecs of the Sun, with the nearest debris disks just a few parsecs away. Debris disks are also important because the secondary material of which they are composed (dust, and at least in some cases gas) can tell us about the composition of exoplanetary material, in a manner analogous to the way in which we learn about the interior of the Earth from asteroid samples and meteorites. Although the evolution of the dust in young planetary systems during the transition from the protoplanetary to debris stage is relatively well understood, understanding the evolution of the gas is a more recent development in the context of debris disks. Arguably the most fundamental question is that of the origin of the gas in debris disk systems: Is it primordial material, indicating that gas and dust evolve on different timescales, or is it second-generation material like the dust component, which might provide insight into the composition of icy bodies in distant planetary systems? A secondary issue is to determine the mass of the gaseous component in order to assess both its importance on the dynamics of solid bodies and the potential for late-stage gas accretion by planets. Deriving the total gas mass in a disk is much more challenging than the dust mass, as uncertain relative abundances and excitation conditions must be considered.
1 point 2. Observations of Debris Disks.
In our Solar System, the zodiacal light appears brighter when observed from Earth, but the Kuiper Belt would appear brighter to a distant alien astronomer owing to the greater mass and larger total surface area of the dust. Neither of the Solar System’s debris disk components would be detectable around a neighboring star using current technology, analogs to the Kuiper Belt are still 1-2 orders of magnitude too faint to be observed with current instrumentation around the majority of stars, and analogs to the zodiacal dust belt are 1,000 times fainter than we can currently detect. All debris disks currently detected around other stars are therefore scaled-up versions of those found in our own planetary system, and we expect that debris disks are far more common than the detected fraction, at least as common as the planetary systems that have been found to be ubiquitous throughout the galaxy. There are many different ways of observing a debris disk. The S-E-D has been the primary diagnostic used to characterize the fractional excess luminosity of the disk over the stellar photosphere, as well as the (range of dust temperature in the disk. Although a simple blackbody excess can be fitted to sparsely sampled S-E-Ds, often multiple components with different temperatures are required when richer data sets are available. With photometric data points in the long-wavelength (submillimeter) tail of the S-E-D, a modified blackbody model that takes into account the decrease in emission, absorption efficiency of small dust grains is used instead. In this case, the long-wavelength slope of the S-E-D is a key observable that can be directly related to the grain size distribution in the disk (see Section 3). Images can reveal the morphology of both gas and dust emission. Dust emission can be imaged across the electromagnetic spectrum to reveal thermal emission as well as scattered light, as illustrated in Figure 2, see also Section 2). In thermal emission, the comparison between the observed disk radius and that expected based on the S-E-D temperature reveals how overheated the dust really is and, thus, the size of the smallest (most populous) grains in the disk. Scattered light images indicate the disk color as well as the degree of forward scattering and linear polarization induced by scattering, again providing constraints on the properties (composition, size, porosity) of the dust grains. Overall, as imaging capabilities have improved across the electromagnetic spectrum, it has become possible to compare the spatial distribution of grains of different sizes within a single disk by making multi wavelength, spatially resolved observations of the disk (see the sidebar titled Observing Wavelength and Grain Size). In addition to the dust, there is occasionally a gas component that can be detected through either emission or absorption spectroscopy. Although early surveys for gas around main sequence stars were impaired by poor sensitivity and low excitation temperature, which led to discouraging low detection rates, a resurgence in interest has followed the development of large collecting area facilities like the Atacama Large Millimeter, submillimeter Array (ALMA). As a result, the study of the gas content of debris disks is now an active and rapidly developing area of study (see Section 4).
1 point 3. Demographics: Detecting Debris Disks.
Our understanding of the demographics of debris disks is driven by large surveys in the Infra Red regime, where the disks are most readily detected through the presence of an Infra Red excess above the stellar photosphere, a signature of circumstellar dust. From their discovery with the Infrared Astronomical Satellite (IRAS) through the Spitzer Space Telescope, AKARI, Wide-Field Infrared Survey Explorer (WISE) and Herschel Space Observatory missions, hundreds of stars have been targeted directly and millions of others observed in all-sky surveys to measure infrared excesses. The rates of incidence typically reported from surveys for debris signatures are in fact better termed detection rates.
Figure 2.
Images of the:
(a) Fomalhaut ring in HST optical scattered light, Herschel 70 micron, and ALMA 1.3 millimeter thermal emission.
(b) Overlay of the 1.3 millimeter ALMA thermal emission map on the HST optical scattered light image of “A-U” Microscopii Panel a adapted from Kalas et al, 2013, panel b from Acke et al, 2012, and panel c from MacGregor et al, 2017. Abbreviations: ALMA, Atacama Large Millimeter Array, FIR, far-infrared, HST, Hubble Space Telescope, mm, millimeter, Vis, visible.
Comparison of detection rates in different surveys is difficult because they often probe different regions of the disk, and the likelihood of detecting a debris disk around a nearby star depends on the wavelength and sensitivity of the observation, the characterization of the photosphere, and the inherent properties of the disk and the stars it surrounds.
Observing wavelength and grain size.
It is often stated that observations of dust thermal emission or scattered light are most sensitive to grains of size approximately equal to the observing wavelength. This correspondence results from a balance between two competing trends. On the one hand, the grain size distribution is heavily weighted toward small grains, which thus dominate the emitting surface area. On the other hand, dust grains only emit efficiently at wavelengths shorter than their physical size, with a steep decline in emission efficiency at longer wavelengths. Therefore, at a given wavelength, only grains comparable with or larger than that wavelength can emit efficiently. As a result, the total (thermal or scattered) light output is dominated by the smallest grains capable of emitting efficiently, namely, grains with sizes approximately equal to the wavelength of observation, if those are present in the system
Many of the results of Spitzer and Herschel surveys to date were detailed by Matthews et al, 2014b, here, we detail primarily new analyses undertaken since that review.
1 point 3 point 1. Kuiper Belt analogs.
Two large surveys of nearby stars for debris disks were undertaken by Herschel, which have yielded consistent detection rates of around 17 to 20 percent for solar-type stars from the DEBRIS and DUNES surveys, comparable with that found at 70 micro meter with Spitzer. Sibthorpe et al further use the properties of the detected disks and the host stars of the DEBRIS survey to gauge the completeness of their detection rates, leading to completeness adjusted incidence rates of 36 percent, 18 percent, 24 percent, and 21 percent for the F, G, K, and total samples, respectively. The F star incidence rate is distinctly higher than that of G and K stars and is in fact more comparable with that of the A star population. This difference suggests that the traditional grouping of all FGK stars into a monolithic solar-type class may not be optimal. Furthermore, there is a significant difference in detection rates between early and late F stars, and a similar but less significant break within K stars. Therefore, for samples dominated by stars in the F5 to K4 range, the variation of detection rate with spectral type is less acute than in samples that extend beyond those boundaries. This conclusion is consistent with the finding of no significant variation with spectral type found by Montesinos et al in 2016, with the Herschel DUNES sample and Sierchio et al in 2014, in a study of F4 to K4 stars based on Spitzer and Herschel data. To date, there remain only a few disks detected around M stars. Whether this is because of the poorer sensitivity in terms of fractional luminosity for M stars or different progenitor properties is not yet resolved. The current detection rates are typically in the range of a few percent. The M star disk population is discussed in the review by Matthews et al, 2014b.
A declining detection rate with age is known and expected for debris disks, because they collisionally evolve over time. For example, Riviere-Marichalar et al 2014, observed 19 members of the 23 Mega year Beta Pictoris moving group with Herschel and found an excess detection rate of around 50 percent. Among compiled data toward several young 20 to 50 Mega year moving groups, Moor et al in 2016 find a much lower rate of detections for K stars (20 percent) versus late F and G stars (56 percent).
Though the rate for the F and G types is markedly higher than that for the older populations measured for DUNES and DEBRIS. These data show that the trend in decreased detection rate as a function of spectral type is real, but it is more difficult to detect at later ages and in some spectral ranges than in others, meaning the gradient of the change may be gradual but have some sharp transitions, particularly at later ages. The multiplicity of a system may lower the rate of detected disks for intermediate spatial separations, but compact or very large disks are detected. Disks may be either circumprimary, or even circumsecondary, or circumbinary as dictated by the separation of the primary and companion.
1 point 3 point 2. Exozodis.
Dust in the terrestrial zone, meaning the warm dust in multiple component disks, see Figure 1, can generally be termed exozodiacal dust, analogous to the 270-K zodiacal dust in the Solar System or to the very hot component known to exist near the Sun.
Exozodis are of interest not just for insight into the underlying planetary systems but also because their presence can significantly hinder our ability to detect planets in the habitable zone. Even Solar System levels of dust, 1,000 times less than the levels we can currently detect, can hinder detection of planets. We refer the reader to Kral et al, 2017b for a recent comprehensive review of exozodis and only briefly discuss recent studies of their occurrence rate. To date, there have been many surveys with space-based single dishes for warm dust emission. These include IRAS, Infrared Space Observatory, AKARI, and, more recently, Spitzer and WISE.
Based on these surveys, the detection rate of warm dust was found to be quite low, in the range of 1 to 2 percent for young stars and up to 100 times lower for older stars. Excesses at mid-IR (MIR) wavelengths can be created by the Wein tail of cold dust. Significantly higher detection rates have been measured utilizing high spatial resolution MIR and NIR interferometry. Mennesson et al 2014 present detections of MIR excesses from the Keck Interferometer Nuller toward 47 stars, finding detection rates above 30 percent for A stars with a global rate of 12 percent. Within this sample, detection of MIR excesses is most common for stars earlier than F2 that also exhibit Kuiper Belt analogs, suggesting that there is a common physical origin for the two belts or that the warm dust belt is sustained by the cold belt through some mechanism. Therefore, the exozodis measured through MIR interferometry are consistent with the warm dust components of two-temperature belts, detected through S-E-Ds or in single dish surveys, albeit at much higher detection rates when these components are observed with sufficient spatial resolution.
Interestingly, MIR excesses have not been detected around stars that host hot dust detected through NIR interferometry. Ertel et al 2014 present a merged sample of 125 stars observed for NIR excesses. Based on their data, detection rates of hot dust decline from 28 percent for A-type stars, to 15 percent for F stars, down to 10percent for G and K-type stars, these values are very similar to the most recent measured rates for Kuiper Belt analogs. The data suggest that the detected rate of hot dust increases with stellar age, which is opposite of what is expected for a collisional cascade and consistent with the idea that the hot dust components are not generated by a steady-state process. For A stars, no obvious correlation is seen between the presence of NIR emission and the presence of a cold Kuiper Belt analog, Nunez et al 2017 note that there is, however, a strong correlation for solar-type (FGK) stars. A correlation with the presence of an outer dust belt is expected, because hot dust cannot be created in situ, and therefore it should be correlated with some outer reservoir of material from which the hot dust is drawn. Modeling of a sample of nine hot exozodis found that the dust was located farther from the star for higher stellar luminosities, so that the dust appears to have a consistent temperature in each system. The dominant source of the flux detected was thermal emission, although the models could not exclude some fraction arising from scattered light. Many of the systems with detected exozodis are old, greater than 100 Mega year, making the origin and high frequency of the exozodis an active area of research. The short timescale required for dust depletion due to collisional evolution or radiative forces is even more acute closer to the star. In steady state, a typically observed quantity of hot dust would survive for only a century: Either the dust is replenished or there exists a means of keeping the dust in situ longer without dissipation. Kral et al, 2017a) describe several mechanisms for moving material inward in a planetary system that could assist in a build-up of dust in the inner parts of a planetary system. For example, Lisse et al 2017, find evidence for hot dust emission in ground-based spectroscopy of HR 4796A’s young debris disk, which has an outer cold component. They find that some of the excess seen in the NIR is due to a tenuous 850-K component with evidence of organics and silicate emission. The authors suggest that that the emission is consistent with a steady stream of dust flowing into the sublimation zone from the disintegration of rocky cometary material.
1 point 4. Scope of This Review.
This review is focused on recent observational developments in understanding debris disk structure, composition, and variability. There have been a number of notable advances in observational capability across the electromagnetic spectrum in recent years that have substantially enhanced our ability to spatially resolve debris disk structure at multiple wavelengths, yielding insight into the physical mechanisms shaping their evolution.
Recent spatially resolved observations from facilities like the Hubble Space Telescope (HST), the Gemini Planet Imager (GPI), the Spectro-Polarimetric High-contrast Exoplanet REsearch instrument (SPHERE), Herschel, and ALMA dominate the major results in this review. We approach the review from the observational direction while pointing out ties to theoretical work, which is intended to be in a complementary direction to other recent reviews.
We have attempted to select topics without comprehensive recent reviews. For example, we have provided in this introduction only a brief update on debris disk demographics, because several recent major reviews have covered that area thoroughly, addressing questions like the incidence of debris disks around stars of different ages and masses, and how debris disk incidence as a function of time connects to planet-formation processes. We also limit the scope of this review to stars on the main sequence, because there are two comprehensive recent reviews of the rapidly developing field of debris disks around post-main sequence stars.
Recently, the field has been progressing from statistics to integrating our knowledge toward understanding of the underlying planetary system and its dynamics. Therefore, in addition to addressing the overall structure (Section 2), dust (Section 3), and gas (Section 4) content of debris disks, we also discuss the topic of planet disk interaction, particularly when informed with direct imaging of both disks and planets (Section 6), and explore the emerging field of time-domain studies of debris disk properties (Section 5).
2. Outer disk structure.
In this section, we review the diversity and properties of spatially resolved structure in outer debris disks, meaning Kuiper Belt analogs. Recent advances in imaging capability across the electromagnetic spectrum have revealed details of disk structure that were previously unobservable and have begun to allow us to piece together a multi wavelength picture that connects the morphology to the underlying physics shaping the disk structure. A spectacular example of such multi wavelength imaging of the Fomalhaut debris ring is shown in Figure 2. Catalogs of resolved images of circumstellar disks are maintained online.
Debris disk imaging efforts stretch back across decades essentially to the moment that debris disks were discovered. We focus on recent observational results, particularly emphasizing disks that have been imaged at a wide range of wavelengths at high, around tens of astronomical units or better, angular resolution. HST, in particular, has been a consistent engine of high-resolution images of debris disks, and we include some recent results that make use of innovative data analysis techniques to draw out better contrast and reduce the inner working angle, meaning, the area of poor contrast near the star created by the coronagraph and related star subtraction post processing techniques.
The diversity of the spatially resolved structure in outer disks can be divided roughly into categories of radial structure, azimuthal structure, meaning departures from axisymmetry, and vertical structure. A summary figure illustrating examples of the different structures discussed is presented in Figure 3. Some disk features do not fall neatly into any category, for example, warping, which generally involves changes as a function of radius that cause discontinuities in the vertical dimension. We review these observations with an eye toward understanding the underlying physical mechanisms driving the diversity observed in the spatially resolved structure.
Figure 3.
Mosaic of scattered light and millimeter thermal emission for eight systems illustrating the range of asymmetries observed in debris disks. In some cases, smoothing and high-pass filtering have been applied to the data to better emphasize substructures.
2 point 1. Radial Structure.
Many debris disks are well described as a collection of rings. Some are narrow, some are broad, and some systems have multiple rings or gaps within an otherwise broad ring. In this section, we explore the diversity of radial structures in debris disks and discuss connections with theoretical mechanisms describing their origin. Figure 4 summarizes the radial extent of all of the debris disks with well-resolved observations, meaning, the angular resolution is small compared to the disk angular extent) at a variety of different wavelengths. The tendency of scattered light observations to yield radially narrower structures is likely due to a selection effect (surface brightness contrast is enhanced in the narrow ring) and possible methodology biases (postprocessing methods to suppress contaminating starlight often partially suppress disk brightness and sharpen existing structures). Also, scattered light images are limited by the inner working angle within which artifacts from subtracting the bright star obscure disk structure. For instance, the inner edge of the solid bar for two disks (HD 15745 and HD 53143) marks the inner working angle rather than a true inner disk edge, because the inner radius is not resolved. Similarly, our detection of the outermost region of each disk is sensitivity limited and thus the full extent of the disks is likely underestimated. Although these systems are all far dustier than our own Solar System’s Kuiper Belt, the radial extent is comparable: The classical Kuiper Belt has an inner radius of 6 Terra-meters, or 40 “A-U” and a width of 1.5 Terra-meters, 10 “A-U”, whereas the scattered belt extends hundreds of astronomical units farther in radius.
Figure 4.
Radial extent of debris disks as determined in scattered light, MIR, FIR, and sub millimeter high resolution observations (blue, gold, orange, and red bars, respectively). Green bars represent the disk structure derived from combined fits to multiple types of data. For each disk and observing methodology, the thick bar and whiskers indicate the FWHM of the inferred surface density profile and the full extent over which the disk is detected, respectively. In most cases, the latter is sensitivity-limited toward large radii, whereas in scattered light, the region inside the main ring is affected by severe artifacts that preclude definitive conclusions. The Solar System’s Kuiper Belt is shown in gray for comparison.
There are no obvious trends in disk radius or disk width as a function of stellar spectral type. Studies of the temperature of the dust from the S-E-D have revealed either weak trends toward a constant temperature regardless of spectral type, possibly due to ice lines setting the disk location, or a slight correlation between dust temperature and spectral type suggestive of an alternative mechanism, but the spatially resolved data have so far not yielded similar trends (although the sample sizes are smaller). In general, the search for trends between disk size and stellar parameters like spectral type or age is used to distinguish between different proposed mechanisms for the dust generation that results in different patterns of radial rings.
Briefly, the proposed mechanisms tend to divide into the following categories: delayed or self-stirring which predicts that dust is generated at larger radii with increasing time, stirring by a planet, in which the radius of the disk can be related to the mass, semimajor axis, and eccentricity of the stirring planet. Or explanations related to ice lines or cometary sublimation.There are also explanations that tend more toward stochasticity but rely on rare events to initiate the collisional cascade, for example, a massive collision or stellar flyby and therefore are unlikely to explain the majority of the extremely commonly observed debris disk population.
For a more detailed description of proposed stirring mechanisms, we refer to Wyatt (2008) and references therein. As Figure 4 shows, the maximum radial extent of debris disks tends to be largest for the most luminous stars and smallest for the least luminous, although in most cases the large outer radius is a scattered light (or IR) feature easily understood as a halo of small grains blown from the parent planetesimal belt by radiation pressure or a stellar wind. These extended halos are common around A-star debris disks, with spectacular examples imaged around Vega and HR 8799. The phenomenon is not limited to A stars, however, for example, the disk around the M star “A-U” Microscopii also exhibits an extended scattered light halo reaching to hundreds of astronomical units distance from the star, whereas the large grains traced by millimeter-wavelength thermal emission are confined within 6 Terra-meters 40 “A-U.” This configuration was explained by the model of Strubbe and Chiang 2006 in which a “birth ring” of parent planetesimals initiate a collisional cascade, the smallest grains of which are then blown out into an extended halo. The surface brightness profile of the halo then reflects the importance of collisions relative to Poynting-Robertson drag. This birth ring paradigm has also been observed in Beta Pic, with a number of other systems exhibiting extended scattered light haloes as well. A few canonical narrow ring systems, including Fomalhaut and HR 4796A, also show faint extended features that may indicate that the phenomenon is widespread at a variety of contrast levels. Another interesting observation is that at least some disks have tenuous dust haloes inside the parent belt, which may be populated by grains that spiral inward due to Poynting-Robertson drag.
Aside from the halo phenomenon, narrow rings, in this case, we mean rings with delta R over R less than about 0.5 are generally quite common, with particularly spectacular examples found around the A stars Fomalhaut and HR 4796A, as well as the F star HD 181327.
There are also examples of radially broad debris disks, even when traced by large grains impervious to radiation pressure at millimeter wavelengths, including those around the A stars HR 8799 and 49 Ceti, the G stars Tau Ceti and HD 107146, and the M star “A-U” Mic.
The question of what makes a ring narrow or broad is still a fairly open one. The broad rings around “A-U” Mic and HD 107146 both exhibit surface brightness profiles that increase steeply with radius at millimeter wavelengths, which is consistent with predictions for self-stirred debris disks, which include planetesimals but not necessarily any planets.
Although the timescale required to assemble Pluto-size planetesimals and initiate a collisional cascade at the outer boundaries of these disks is longer than the age of the systems. The 49 Cet disk, by contrast, exhibits a deficit of millimeter emission at small radii, with a surface density profile that generally decreases with radius beyond around 15 Terra-meters, 100 “A-U”, extending to radii of more than 45 Terra-meters or 300 Astronomical Units. Given similar difficulties with reconciling the age of the system with the timescale for self-stirring at such large radii, it is likely that at least some parts of the disk are stirred by planets or other mechanisms.
Narrow rings, however, may require multiple planets shepherding the inner and outer radii of the ring or truncation by external perturbers, or they may be confined by mechanisms related to the interaction between gas and dust. Substructure in the radial dust distribution is now becoming visible as high-contrast imaging techniques improve.
For example, the disk around HD 07146 contains a broad and smooth ring at optical wavelengths, but is much broader and exhibits a statistically significant gap in its radial brightness distribution at millimeter wavelengths. Similarly, the disk around HD 131835 shows clear evidence for multiple rings, as does that around HD 120326. A related phenomenon, veering into the territory of departures from axisymmetry that is discussed below, is that of arcs and spiral arms, which have been imaged in the disks around HD 141569 and HD 53143. HD 141569 may be unique in representing a truly transitional object between the protoplanetary and debris disk phases, which might explain why it is so unusual in exhibiting a spiral pattern, see Figure 3i, but clearly arcs and concentric rings are a relatively common phenomenon in debris disk systems.
At this point the data quality is not sufficient to distinguish between a broad disk punctuated by narrow gaps and multiple concentric narrow rings in most cases, though recent high-resolution ALMA observations of the HD 107146 system are extremely suggestive of a gap-like structure that retains dust at a reduced level within the gap. Such a broad and shallow gap is likely to be more consistent with planet-sculpting scenarios than gas-dust interactions, and in debris systems these gaps are quite easy to open and might be sensitive to very low-mass planets. As a final example of radial substructure, we must consider the presence of warps in debris disks. The iconic example within this category is the disk around Beta Pic, which exhibited a clearly warped inner disk in early scattered light imaging that pointed the way to the planet Beta Pic b. Similar structures have been observed in the disks around “A-U” Mic and HD 110058. Interestingly, modeling suggests that a single planet may produce only transient warps, and may not be able to explain all of the observed features of the Beta Pic system, indicating that multiple planets or other mechanisms may be necessary to sustain warped structures.
2 point 2. Departures from Axisymmetry.
With the central star providing an essentially spherically symmetric radiation field and acting as a point mass, departures from axisymmetry are of particular interest because they require something beyond the star-disk system to break the axisymmetry. One tempting interpretation is gravitational sculpting by a planet, but other interpretations have also been proposed for various phenomena, including interactions with the I-S-M, gravitational perturbations from stellar flybys or companions, instabilities produced by interactions between gas and dust, and recent planetesimal collisions. Here, we review several categories of nonaxisymmetric structure in debris disks.
2 point 2 point 1. Eccentricity.
A number of debris disks exhibit subtle deviations from axisymmetry in the form of eccentricity, such as Fomalhaut. Nearly all narrow rings have a stellocentric offset. Measured eccentricities range from around 0.06, HR 4796A, HD 15115, to around 0.2 for HD 106906. Eccentricities smaller than around 0.05 cannot routinely be measured with current instrumentation. A detailed look at the flux distribution in an eccentric disk provides a wealth of information about the grains and their dynamics. In scattered light observations at short wavelengths, debris disks exhibit a brightness enhancement at pericenter due to the steep one over r squared drop off in flux with distance from the central star, the closest point to the star (pericenter) therefore glows the brightest. At longer wavelengths, by contrast, thermal emission in an optically thin disk is proportional to the product of temperature, which decreases as one over the square root of r with distance from the star, surface density, and opacity.
In the MIR to far-infrared (FIR), the higher temperature at pericenter can result in pericenter glow, whereas in the submillimeter, the accumulation of dust grains on eccentric orbits at apocenter leads to an opposite brightness asymmetry, called apocenter glow. Both pericenter and apocenter glow are observed in the Fomalhaut ring at different wavelengths (see Figure 2 b, c). The variation of the apo-to-peri flux ratio as a function of wavelength can be related, in the context of steady-state models of grain populations, to properties of the grain size distribution like its power-law slope q and the long-wavelength slope of the grain emissivity Beta. Recent high-resolution ALMA imaging of the Fomalhaut system has provided the first conclusive observational evidence for apocenter glow and has been used to place stringent constraints on the power-law slope of the grain size distribution. Detailed models of the scattered light from the HD 61005 disk perplexingly derive a density that is two times higher at pericenter than apocenter. The underlying cause of eccentric rings in debris disks is more difficult to pinpoint. The categories are similar to those proposed as the confinement mechanism for narrow rings, although eccentricity requires mechanisms that break the intrinsic symmetry of the star-disk system, meaning, eccentric planets, dust-gas interactions, and recent catastrophic giant impacts. A potential way of distinguishing between a recent collision and a more steady-state planet shepherding scenario is via the long-wavelength slope of the S-E-D, because impacts are predicted to exhibit specific particle size distributions.
2 point 2 point 2. Swept-back wings.
Another departure from axisymmetry is the asymmetric, sweptback features occasionally associated with dust rings in debris disks. The prototypical example is HD 61005, also poetically named “The Moth” owing to the appearance of the swept-back “wings” that dominate its scattered light images (see Figure 3h). Also included in this category are HD 32297 and HD 15115, and two other possible members are the asymmetric edge-on disks around HD 111520 and HD 30447. Schneider et al in 2014 also note that systems like Fomalhaut and HR 4796A that exhibit low signal-to-noise ratio (SNR), which is evidence of faint extended features beyond a narrow dust ring, may indicate that these systems are simply the most spectacular examples of a common phenomenon, perhaps due to their edge-on configuration. As a class, in addition to their large-scale asymmetries, these systems feature a distinct ring component, which may be eccentric as in the case of HD 61005 or apparently circular as in the case of HD 15115, and color gradients that trace the structural asymmetries in scattered light. The degree of asymmetry of these systems in early millimeter interferometry mapping is weak if present at all but higher-resolution mapping with ALMA is crucially needed to assess whether large dust grains trace the asymmetry observed in scattered light or are primarily confined to the parent-body ring.
The most popular explanation for this morphology has so far been interactions with the I-S-M. Two similar models based on interaction between disk particle and I-S-M gas under different configurations have been proposed to explain the observed structures. Another torque-based method of breaking the symmetry, proposed by Esposito et al in 2016, involves an eccentric, inclined planet sculpting the dust in the HD 61005 system. Independently, Lee and Chiang 2016 developed a unifying model of debris disk morphology that invokes planets to explain a wide range of morphological features including needles and wings like those observed in HD 15115 and HD 61005. To provide yet another interpretation, Mazoyer et al in 2014 speculate that a recent collision like that described by Jackson et al in 2014 might plausibly explain the morphology of HD 15115.
At this time, no definitive explanation for the observed structure exists, although higher-resolution imaging at long wavelengths that spatially resolves the locations of the large grains is one promising avenue of investigation, because I-S-M interactions generally preferentially manifest in the small grains, whereas dynamical scenarios are more likely to affect the large grains as well.
2 point 2 point 3. Clumps.
Clumpy structure in debris disks has long been a predicted consequence of resonant interactions between planets and debris dust. The prediction is reasoned largely on the basis of analogy with Neptune and the resonant structure it induces on Kuiper Belt objects (KBOs) in our Solar System. The predicted orbital period of a dusty clump is super-Keplerian, orbiting with the period of the planet inducing the resonance rather than at the orbital period of the more distant KBOs themselves. Such features are most readily observed at long wavelengths that trace large dust grains, because the radiation pressure that affects the orbits of small grains can smooth over and erase clumpy structure. Although initially there appeared to be clumpy structure in low SNR millimeter-wavelength maps of several systems, including Vega and epsilon Eridani, follow-up observations have generally not robustly recovered the clumpy structure. There are different explanations for different sources: In the case of Vega a combination of low SNR and positioning of the features at the edge of the primary beam where noise is difficult to characterize likely led to an overestimate of the significance of the reported clumpy structures.
In epsilon Eridani, by contrast, while it is still not clear whether the features are recovered or not, it is clear that background galaxies account for at least some of the previously reported clumpy structure, because the high proper-motion system recently passed in front of an unusually strong concentration of galaxies bright in the submillimeter.
At this stage, the only system with an unambiguous departure from axisymmetry in the form of a “clump” of millimeter emission on one side is the debris disk around Beta Pic, which exhibits a relatively weak asymmetry in dust continuum emission and a stronger asymmetry in Carbon Monoxide emission see also Figure 3g. Unfortunately, because Beta Pic is viewed edge-on, it is impossible to study the true azimuthal variation of the dust emission. In general, even with highsensitivity imaging by ALMA, Beta Pic has so far been the primary exception to the rule that debris disks tend to be extremely azimuthally smooth at millimeter wavelengths.
A robust approach to determining the role of low SNR features is to subtract an axisymmetric model and examine the residuals, in the visibility domain, for interferometric data, but the role of background galaxies is much more difficult to determine without long-term observations that monitor changes in structure because of both orbital motion in the system and proper motion of the system across the sky. The low levels of nonaxisymmetry do not rule out the presence of planets.
Despite the earlier predictions of resonant structure, recent sophisticated modeling of debris disk evolution that takes into account the role of collisions shows that resonant structure can be washed out by collisions even for the large grains that dominate the emission at millimeter wavelengths. Another implication of these models is that in systems with lower dust densities in which the collision rate is proportionally lower, collisions may be less efficient at washing out the signature of resonant interactions. Shannon et al in 2015 present a fast, semianalytic method for generating images of such (collisionless) disks for comparison with data.
Asymmetries are also expected to be more common in the terrestrial planet-forming regions, where velocities are large and destructive collisions between planetary embryos more frequent. Another potential method of distinguishing between planet and non planet mechanisms is studying the alignment of the disk’s spin axis with the parent star’s rotation axis, which can be connected to planetary obliquity studies. Although this field is still in its infancy, some initial investigations show that the rotation axes of disks and stars are mostly well aligned.
2 point 3. Vertical Structure.
The vertical structure of debris disks can be a revealing probe of the physical mechanisms shaping debris disk structure. Unfortunately, due to degeneracy between the vertical structure, radial structure, and viewing geometry in optically thin disks seen at intermediate inclinations, it can be difficult to measure. The most favorable, albeit still imperfect, case for measuring the vertical structure is an edge-on, radially narrow disk, but in scattered light images, point spread function subtraction can complicate the interpretation of the disk width. There have been several measurements of vertical structure in debris disks in this regime. The vertical structure inferred from image modeling is generally expressed as an h over r ratio, where h is the scale height, typically Gaussian sigma or HWHM, half width at half maximum, of a Lorentzian or other distribution, and r is the disk radius.
An interesting apparent trend, from the few disks with sufficient resolution to make such a determination, is that these systems exhibit a projected FWHM, full width at half maximum, perpendicular to the disk major axis that is constant or decreases with radius close to the star and then switches direction to increase with radius far from the star, possibly with the outer edge of the parent planetesimal belt falling somewhere near the inflection point. This is best seen in the case of “A-U” Mic and Beta Pic. The behavior inside of the parent body projected radius likely stems from the combination of a radially broad parent body belt with a (more or less) forward-scattering phase function. The sharp increase outside of this radius is an effect of the radial-versus-vertical degeneracy applied to prominent haloes in these systems. The most reliable estimates of the scale height are obtained for systems in which the scattered light has been modeled within a framework that takes into account the phase function, rather than systems in which only the projected FWHM on the sky is recorded, because the projected FWHM is degenerate with the inclination of the system. Such modeling has been carried out for a half-dozen disks so far, with h over r values ranging between 0.02 and 0.12 with a median of 0.06. The vertical structure of debris disks has often been interpreted as a measurement of dynamical excitation, indeed, it is the most direct way of probing the system’s velocity dispersion and, therefore, the processes and masses of the bodies sculpting it. The large bodies stirring the collisional cascade gravitationally excite the small dust grains, increasing their eccentricity and inclination through collisions that bring the bodies into equilibrium with impact velocities comparable with the escape velocity of the largest bodies. It is therefore possible to relate the disk thickness directly to the mass of the largest bodies stirring the collisional cascade. However, Thebault in 2009 demonstrates that the vertical structure at optical and Infra Red wavelengths should exhibit a substantial “natural” scale height of 0.04 plus or minus 0.02 due to radiation pressure from the star that can excite the eccentricities and inclinations of the small grains that dominate the scattered light images, even in the absence of large bodies. Therefore, caution must be exercised in interpreting these scale heights as probes of the dynamical state of host debris disks.
The high angular resolution and sensitivity available with ALMA provides an opportunity to circumvent this problem by measuring scale heights at longer wavelengths, in which the grains that dominate the thermal emission are large enough to be effectively impervious to the radiation pressure that produces the natural scale height at optical and Infra Red wavelengths. There is even some evidence from multiwavelength dynamical modeling of the S-E-D and resolved images that the “A-U” Mic system should display a smaller scale height at millimeter wavelengths than at shorter wavelengths. Self-consistent modeling of the size-dependent velocity distribution in the collisional cascade by Pan and Schlichting in 2012 allows vertical scale height measurements at a particular (millimeter) wavelength to be connected with the dynamical state of the debris disk in a robust way.
Three. Dust properties.
In this section, we discuss how observations of debris disks are used to place constraints on some key dust properties, such as composition and size distribution. The morphology and S-E-D of a debris disk are jointly determined by its geometry and by the properties of the dust grains it contains. Although some results are solidly established, we also emphasize some issues that are currently open but where progress is possible in the future.
3 point 1. From Observations to Dust Properties.
The absorption, emission, and scattering properties of a dust grain depend on its physical characteristics, size, shape, composition, porosity. These quantities can be modeled parametrically or with theoretically grounded models. Although the latter are preferable to illuminate the origin of the debris disk phenomenon and its role in the evolution of planetary systems, they are generally more challenging to implement and often rely on assumptions that are difficult to verify. We first present a brief overview of both types of models before describing how physical parameters qualitatively affect the appearance of debris disks.
3 point 1 point 1. General methodology.
The thermal emission of debris disks is determined by the balance between the heating (absorption) and cooling (emission) of dust grains. The fact that small dust grains are imperfect long-wavelength emitters results in hotter-than-blackbody dust temperatures, as well as in a long-wavelength S-E-D tail that is steeper than the Rayleigh-Jeans law. Analyses of debris disk S-E-D’s generally adopt the modified blackbody model to represent this behavior (see the sidebar titled Modified Blackbody Models). A scattered light image provides constraints on several quantities: albedo, color, scattering phase function, and polarizability. The latter two quantities measure the dependence of scattered intensity and linear polarization, respectively, on scattering angle. A resolved surface brightness map can therefore be used, under the assumption of axisymmetric volume density, to derive the dust scattering phase function.
Modified blackbody models.
The fact that small dust grains are imperfect long-wavelength emitters is most often described with a two-parameter opacity law, whereby the perfect blackbody emission is multiplied either by a lambda to the power of minus Beta term for lambda greater than or equal to lambda zero, after Backman and Paresce 1993, or by one minus e to the minus lambda zero over lambda to the power of beta, after Williams et al 2004. The latter formalism asymptotically converges to the same power law at the longest wavelengths as the former while avoiding its sharp break around lambda zero.
HENYEY-GREENSTEIN SCATTERING PHASE FUNCTION.
The most commonly used analytical prescription for the scattering phase function is the Henyey-Greenstein formalism, Henyey and Greenstein 1941, which is described by a single parameter, the asymmetry parameter g, and is the intensity-averaged cosine of the scattering angle. In this prescription, the scattered intensity varies as a function I of theta, see text, where θ is the scattering angle. Isotropic scattering corresponds to g equals zero, whereas forward and back scattering are characterized by positive and negative values of g, respectively. Although it is easy to implement, this formalism does not rely on any physical model of scattering. Furthermore, empirically determined scattering phase functions generally have a significantly different shape, which can at best be approximated by the linear combination of several distinct Henyey-Greenstein phase functions. Interpreting scattered light images of astrophysical objects with this formalism can therefore be misleading, as discussed in this review for debris disks.
Although the dust albedo can in principle be used to derive the grain composition, it relies on knowledge of the Bond albedo, averaged over all scattering angles, which is not accessible from our fixed vantage point. However, the scattered light color of a disk relative to its parent star is essentially a differential albedo measurement and is therefore more directly informative about the dust properties.
The most common analysis tool for scattering phase functions is the analytical Henyey-Greenstein prescription, characterized by the asymmetry parameter g (see the sidebar titled Henyey-Greenstein Scattering Phase Function). One practical complication is that estimating the sign of g requires identifying which side of the disk is in front of the parent star, which is normally assumed to be the brightest as a result of preferential forward scattering, but can be ambiguous in some cases. Other approaches involve using Mie theory for spherical grains or numerical approximations applicable to complex grain shapes.
Because starlight is unpolarized while scattered light is polarized, imaging polarimetry with instruments such as GPI and SPHERE provides a powerful analysis tool. The simplest parametric model for polarizability is Rayleigh scattering, even though it is unlikely to be physically appropriate for the micron-sized (and larger) dust grains found in debris disks. As a result, a combination of Rayleigh polarizability and Henyey-Greenstein phase function is sometimes adopted.
Although each of the approaches discussed above provides important clues regarding the dust properties of a debris disk, they all suffer from ambiguities and potential biases. Critically, while parametric models are easy to implement, they do not rely on a physical framework and their interpretation in terms of dust properties is not unique. A physically grounded, multi wavelength approach that combines several methodologies is required to obtain a complete picture of the dust properties. Such analyses are based on Mie theory, as this is the only theoretical framework that provides a self-consistent estimate of the absorption, scattering, and emission cross-sections at all wavelengths. The key parameters in such a model include the minimum grain size, the power-law index of the grain size distribution, the dust composition, and the grain porosity, although some of these may be fixed to default values for computational expediency.
3 point 1 point 2. Qualitative behavior.
Having defined the main observational properties of dust populations, we now address how these are affected by key physical properties of the dust grains, such as their composition and size, using the available modeling frameworks.
3 point 1 point 2 point 1. Dust composition and porosity.
In thermal emission, the dust composition is most readily probed through the presence and shape of solid-state spectral features. Most prominent is a collection of silicate features that are observed at wavelengths ranging from 10 to 70 micron, although these are only excited for grains larger than around 10 micron and heated to a minimum of 150 to 200 K, except for the 69 micron forsterite feature, which can be present at lower temperatures. Thus, studies of the silicate mineralogy are limited to small grains in the warm and hot components of debris disks. Within these constraints, many species of silicates can be identified in both amorphous and crystalline forms, with the latter typically displaying sharper features. Besides silicates, many nonsilicate species have spectral features in the MIR, albeit usually weak and, or very broad ones. High SNR spectra, together with dedicated spectral decomposition codes can thus be used to infer their presence in debris disks.
By contrast, dust composition does not significantly affect the modified blackbody Beta parameter.
In scattered light, dust composition primarily influences the surface brightness and color of debris disks. However, even the difference between low-albedo carbon-rich dust and high-albedo silicate and ices-rich species is nearly impossible to establish because the albedo is degenerate with the total mass of scatterers for optically thin disks. In contrast, certain species have markedly distinct colors, such as the organic compounds responsible for red colors observed for many Solar System minor bodies. Finally, high grain porosity can be inferred from (polarized) scattered light imaging, as the first-order effect of porosity is to reduce the effective size of dust grains. In other words, larger porous grains have scattering properties that are qualitatively similar to those o
-
13:43
Cooking with Gruel
2 days agoCreamy Saffron Risotto
24.9K9 -
18:38
DeVory Darkins
18 hours ago $6.94 earnedTrump Makes HUGE Announcement that may spark GOP Battle
19.7K33 -
2:13:05
The Nerd Realm
4 hours ago $1.26 earnedNew Years Eve! Fortnite Hunters w/ YOU! Creator Code: NERDREALM
14.7K2 -
LIVE
FusedAegisTV
19 hours agoNYE Eve! - 2025 Incoming 🎉 - 12hr Variety Stream!
154 watching -
1:18:52
Awaken With JP
6 hours agoSomehow The World DIDN’T End This Year! - LIES Ep 72
52.5K27 -
1:19:34
Michael Franzese
4 hours agoWhat 2024 Taught Us About the Future?
60K17 -
1:48:09
The Quartering
5 hours agoBird Flu PANIC, Sam Hyde DESTROYS Elon Musk & Patrick Bet David & Woke Witcher?
78.6K50 -
4:47
SLS - Street League Skateboarding
3 days agoLiz Akama’s 2nd Place Finish at SLS Tokyo 2024 | Best Tricks
23.1K3 -
LumpyPotatoX2
4 hours agoHappy New Year Rumble ! - #RumbleGaming
15.5K -
10:37
One Bite Pizza Reviews
1 day agoBest of Barstool Pizza Reviews 2024
45.7K29