Premium Only Content
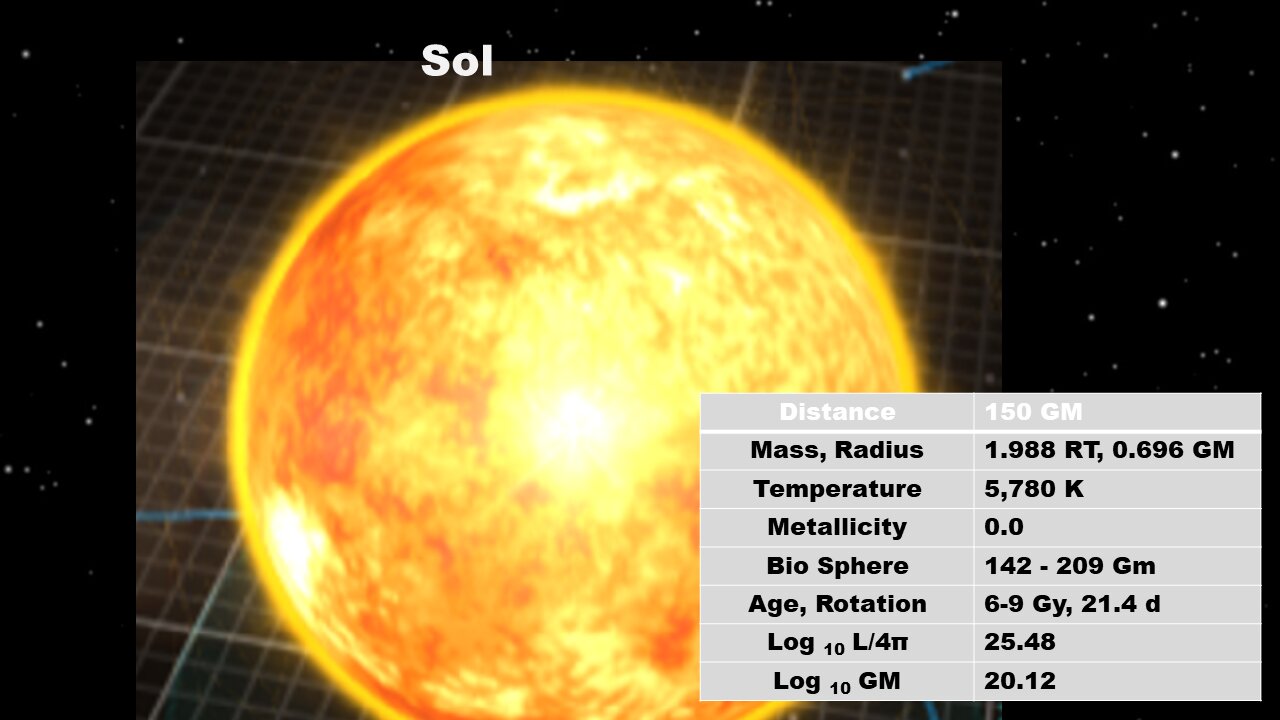
The ten nearest stars. In space, no one can hear you puke.
The ten nearest stars.
Sol.
The Sun.
Sol and its system will be used to introduce the units. Often properties of nearby stars are listed in fractions of solar units, such as luminosity, or solar mass.
The quoted effective stellar temperature refers to the temperature of the outer photosphere. By definition the photosphere is the outer surface of the star through which half of the light escapes un-scattered by collisions with matter. The photosphere of Sol is approximately 100 kilometers thick, and has a density of zero point three milligrams per cubic meter. The temperature in the photosphere is between 4500K and 6000 K, with the effective temperature stated as 5772 K. In the photosphere, bubbles of plasma, or granules, rise, cool and fall back into deeper depths. Each granule is around one thousand kilometers in size.
The spectral classification of a star is often reported in the Morgan-Keenan, MK system, with O being the hottest, brightest and having the shortest lifespan, and M being the coolest, smallest, and longest persisting. Sol, of spectral type G, is a star with an effective temperature between 5200 and 6000 degrees Kelvin. The sub-categorization of “Two”, or G-Two means that the temperature is around 5770K, with a G-One having a temperature of 5860 K, and a G-three a temperature of 5720K. The luminosity class of “V”, often referred to as dwarf means that the star is on the Main-Sequence, and is consuming hydrogen to create Helium. As the Sun ages, the amount of Helium will increase. Currently the ratio of Hydrogen to Helium in the sun is around three to one.
Hence, by the definitions, Sol is a spectral class G two dwarf star, with a photosphere temperature of 5772 Kelvin.
Sol has a mass of 1.99 Rona tons, RT, or 1.99 times ten to the thirty Kilograms, and a radius of 0.969 Giga meters.
Sol is 4.6 Giga years old, and has an equatorial rotational period of 24 point 47 days. As stars are not solid, the rotational period decreases towards the poles, which can result in differential shearing of the atmosphere.
The average orbital period around the Galactic core is two hundred and forty million years. In its lifetime, the Sun has orbited the Galactic center just over nineteen times.
The composition of a star is often reported as its metallicity, which is the ratio of number of Iron atoms to the number of Hydrogen atoms. For the Sun, the ratio of Iron atoms to Hydrogen atoms is about one to twenty thousand.
Expressed as a logarithm, the solar metallicity Log ten of Iron to Hydrogen is minus 4.33.
The metallicity ratio of Iron to Hydrogen for a star, is the log to base ten of the Iron to Hydrogen ratio, minus the log to base ten of the Iron to hydrogen ratio of the Sun. Expressed as a ratio, the logarithmic metallicity Iron to Hydrogen of the sun is Zero.
A metallicity of plus one represents ten times the Iron to Hydrogen of the Sun. A value of minus one corresponds to one tenth of the Iron to hydrogen ratio of the sun. And a value of zero indicates that the star has the same ratio of Iron to Hydrogen as the sun.
An additional specification of stellar composition is the fraction of Hydrogen, Helium and other items.
Fractional hydrogen is denoted as X, fractional Helium is labelled as a Y, and all other elements are denoted as Z, with the sum X plus Y plus Z equal to one. For the Sun, X, the fractional Hydrogen is zero point 70. Y, the fractional Helium is zero point 28, and Z, all other elements are zero point zero two.
The 2015 Resolution B2 of the International Astronomical Union defined a standard luminosity, the zero point luminosity L zero of 3.0128 times ten to the 28 Watts, or approximately 78.7 times the luminosity of Sol.
Minus two point five times the logarithm, of a stars luminosity over this standard zero point value is now known as the bolometric magnitude of the star. For the sun, this bolometric luminosity is 4.74, and is a dimensionless number.
The absolute magnitude of a star is the apparent luminosity of the star if it was viewed at exactly ten parsecs, 30.857 Peta-meters, without scattering and absorption of light by any interstellar material.
The visual absolute magnitude, M V, considers only the light emitted in the visible spectrum. For Sol M V is the dimensionless number 4.83.
An alternative unit introduced here is the “LILO”, or logarithm to base ten of the total solar isotropic luminosity L, which is the bolometric or integrated emitted energy over all frequencies in Joules, divided by four pi. LILO is just the log to base ten of the Radiant Intensity, or logarithm of the Watts per steradian.
For Sol the LILO is: Log L divided by four pi equals 25.484. As an example of the use of LILO, at a distance of ten Giga meters, the logarithmic fluence is 25.484 minus twenty, or 5.484, or about three hundred kJ per square meter.
The standard gravitational parameter is the Cavendish gravitational constant G, times the mass, usually denoted by a Greek letter mu. The Log to base ten of mu, which is log to base ten of G, M, for the sun is 20 point 12.
In units of Giga-meters, the semi-major axis of Sol’s inner planets are located at 58, 108, 150 and 228 giga-meters.
The major outer planets are located at 778, 1,433, 2,871 and 4,500 Giga meters.
The Habitable zone of a star is the range of distances where the surface temperature of planets will allow liquid water. The inner habitable zone of the solar system is zero point nine five “A-U”, or 142 Giga-meters. The outer habitable zone is one point four “A-U”, or 208 Giga meters. These distances will increase or decrease with the square root of the luminosity of the star relative to the sun. Alternatively, using the LILO of the star, the log to base ten of the distances in meters can be determined.
As the star ages, the luminosity will increase. Since the inner radius of the habitable zone is zero point nine five “A-U”, a ten percent increase in luminosity of the sun will place the Earth at the boundary of the habitable zone. At the suns current rate of hydrogen burning, this will occur in approximately one billion years.
Conventionally star distances are given in one of two units. Light years, the distance light can travel in one Julian year of 365 point 25 days. The other unit is the parallelax-seconds, or “Parsec”, derived from one Astronomical Unit, the distance from the Earth to the sun, divided by one second of arc, or a thirty six hundredth of a degree. A Parsec is approximately 3 point 26 light years.
One light year is 9.45 times ten to the fifteen meters, or slightly less than ten Peta-meters. One Peta meter is ten to the fifteen meters, or a million Giga-meters. Ten Peta-meters equals one point zero five light years. Distances are here quoted in Peta meters.
Many of the extra solar planets discovered, were detected using the radial velocity method. Since the star and the unseen planets revolve about their common barycenter, there is a Doppler shift imparted upon the spectral lines of the parent star.
One form of the equation for the radial velocity K, measured in meters per second, is given by Wright, in his 2017 paper, included in the references. The constants are numerical values, and the Gravitational constant G. The system specific values include the eccentricity of the orbit, usually zero to a half, the Period of the rotation P, and the mass of the parent star.
And the variable, “Em-sine-eye”, or the mass of the planet times the sine of the angle of inclination to the observer. Hence if a planet was inclined with an inclination angle of zero, the radial velocity measurement would not be able to detect the planet.
It must also be noted that the radial velocity shift can be quite small. The 2023 limit is a motion of a system of around one meter per second. The motion of the Earth on the sun is 9 centimeters per second, which would not be detectable with 2023 technology.
By comparison, the Jovian scale planet of zero point four Jovian masses around 51 Pegasi b, with a 4.2 day orbit imparts a radial velocity of around 60 meters per second, and a correspondingly large Doppler shift.
With a slight rearrangement of terms, the equation for the Doppler velocity K can be written as
K is:
Cube root of two pi G over M star squared, which are the star specific parameters, times:
M planet over cube root of period P, times the square root of one minus eccentricity,
Which are the planet specific terms, and sine inclination I, which is the observer specific term.
In practice the combination of the planet mass and inclination, “Em-sine-Eye” is usually the determining factor for the detection of an extrasolar planet.
Typical results, from Hurt 2022 for GJ 411, also known as Lalande 21185, show the radial velocity perturbations of planets after processing.
Alternative methods of extra-solar planet detection include transits of a planet across a star. And the Kepler space telescope was designed explicitly for this purpose. In this case, the difference on measured light intensity as the planet occludes part of the star is required, so non-transiting planets cannot be detected.
Direct imaging of a planet can be obtained by image processing of stellar images. By subtracting the optical light, the scattered infra-red can display the position of orbiting planets. Processed images of GJ 504, also known as 59 Virginis, a G zero star about 570 Peta-meters from Sol, from 2011 to 2012 are shown, to display GJ 504 b.
Alpha Centauri.
The Alpha Centauri system consists of three stars.
Alpha Centauri “A”, and Alpha Centauri B, and Proxima Centauri, gravitationally bound with the binary system.
Alpha Centauri “A” is a G Two of 4.34 absolute magnitude at a distance of forty one point six Peta meters from Sol.
Alpha Centauri B is a K Zero, 5.7 absolute magnitude star, revolving at 3,570 Giga-meters from Alpha “A”.
Proxima Centauri is an M Five point 5 of absolute magnitude 15.45. It orbits Alpha Centauri at a distance of 1.5 Peta-meters.
Alpha “A” has of Mass of 2.15 Ronna tons, a Radius of 0.85 Giga-meters, and a Temperature of 5790 Kelvin. The Fe over H Metallicity of 0.2. Its LILO is 25.66, and its Log mu is 20.16. It is very similar to Sol.
The age of Alpha Centauri “A” is 5.17 Giga years, and its rotation period is 22 days.
Alpha “B” has a Mass of 1.81 Ronna tons, a Radius of 0.60 Giga-meters, and a Temperature of 5260 Kelvin. Its Iron to Hydrogen Metallicity is 0.21. Alpha Centauri “B” has a LILO of 25.181, and a Log GM of 20.08.
Alpha Centauri B is 2.53 Giga-years old, with a rotation period of 41 days.
Proxima’s details are that it has a mass of 0.24 Ronna tons, a Radius of 0.11 Giga-meters, a temperature 2879 K, and its Metallicity Iron to Hydrogen is 0.1. Proxima is also a flare star of type BY Draconis.
Proxima Centauri has an age of 4.85 Giga-years and a rotation period of 92.1 days.
The orbits of Alpha and Beta Centauri and Proxima are at an inclination to an observer from Earth, so the Orbit can be readily distinguished through a telescope.
Proxima’s orbit around the alpha and beta Centauri barycenter has an eccentricity of 0.5, with a semi major axis of 1,300 Terra-meters, with an orbital period of 547 thousand years.
Proxima Centauri has three confirmed planets, Proxima B, C and D.
Proxima D has a mass of 0.26 of Earth, and its orbital parameters are: semi-major axis. 4.32 Giga-meters, eccentricity 0.04, period 5.122 days.
Proxima B has a mass of 1.07 of Earth, and its orbital parameters are: semi-major axis 7.27 Giga-meters, eccentricity 0.109, period 11.184 days.
Proxima C is disputed, but would have a mass of seven Earths, and its orbital parameters would be: semi-major axis 222 Giga-meters, eccentricity 0.04, period 1928 days.
“B-Y” Draconis flare stars have flares driven by sunspots and stellar rotation. The prototype is “B-Y” Draconis “A” and “B”, two stars fifty three light years away in the constellation of Draco.
Flare periods are quasiperiodic with frequencies close to the stellar rotation period, with typical amplitudes of approximately half a visual magnitude. The flare oscillations are likely the interplay of the stellar magnetic field and the turbulent surface convection.
Flares which increase the local temperature up to 8000K are known, which can increase the luminosity by up to a half a visual magnitude. Energy fluxes can reach ten to the twenty two Joules of UV or X ray energy.
Bernard’s Star.
Bernard’s Star is an M five, of absolute magnitude 13.24, located at a distance of 56.2 Peta-meters. The Mass of Bernard’s Star is 0.32 Ronna-tons, its Radius is 0.13 Giga-meters, and it has a Temperature of 3,278 K.
Bernard’s star is a “B-Y” Draconis type flare star with the designation of V2500 Ophiuchi.
Bernard’s stars age is 10 Giga-years, and its rotational period is 130.4 days. Bernards Star is smaller, cooler and older than the sun, with an Iron to Hydrogen metallicity of minus 0.12 Dex.
LILO 23.015, Log GM, 19.33.
As yet there are no positively confirmed planets orbiting Bernard’s Star, and the sensitivity of the studies would require any undetected candidates to be approximately earth mass or less.
For example, see the paper by Jack Lubin, 2021: “Stellar Activity Manifesting at a One Year Alias Explains Barnard b as a False Positive”.
Also Artigau, 2022, in “Line-by-line velocity measurements, an outlier-resistant method for precision velocimetry” stated: We confirm with measurements spanning 2.7 years that the candidate super-Earth on a 233-day orbit around Barnard’s star is an artifact due to a combination of time-sampling and activity.
Wolf 3-5-9.
Wolf 3-5-9 is an M six of absolute magnitude 16.56, located at 73.8 Peta-meters.
Wolf 3-5-9 has a mass 0.22 Ronna-tons, a Radius 0.10 Giga-meters, and an effective Temperature of 2,749 K. Because of its small size, Wolf 3-5-9 is fully convective, so that the internal material physically cycles through the star, maintaining the spectral type for a considerable time period. Wolf 3-5-9 is likely to remain a main sequence for two trillion years.
Wolf 3-5-9 is a young star, between one hundred to three fifty million years old. It is a UV Ceti type flare star with the fares driven by magnetic activity in the atmosphere. Such flares can be quite short, in terms of minutes. The magnetic field of Wolf 3-5-9 is around 0.2 Tesla’s, and can vary over times scales of hours. Flares can be around ten to twenty percent of the size of the star, compared to the few thousand for solar flares. X ray luminosities can be up to one percent of the bolometric luminosities. Flares of two magnitudes of luminosity have been observed.
Wolf 3-5-9 rotates once every 2.70 days, and has a metallicity of 0.18, a LILO of 22.509, and a Log GM of 19.16.
The candidate planets of Wolf 3-5-9 consist of Wolf 3-5-9 B and C. Wolf 3-5-9 B has a predicted mass of around forty four Earths, with an orbit radius of 275 Giga-meters. The existence of Wolf 3-5-9 C is dubious, but it would have a mass of around four Earths, with a period of two point seven days, and an orbit radius of just over seven Giga-meters.
Lalande 21185.
Lalande 21185 is an M two of absolute magnitude 10.46, at a distance of 78.6 Peta-meters.
Mass 0.77 Ronna-tons, Radius 0.27 Giga-meters, and a Temperature of 3547 Kelvin. Lalande 21185 is a “B-Y” Draconis type variable star, with the variable star designation NSV 18593.
Lalande 21185 is 5 to 10 Giga-years old, and rotates once every 56 days.
Its metallicity, Iron to H, is -0.09.
LILO 23.774, log ten GM 19.71.
Lalande has three candidate planets, located at 11.8, 76.6 and 424 Giga-meters.
Only one of the planets of Lalande 21185 is confirmed, Lalande 21185 b, with an orbital period of 12.93 days. Lalande 21185 b has the orbital elements of eccentricity 0.12, Semi major axis 11.8 Giga-meters, and an orbital period of 12.9 days. Estimated equilibrium temperature is 370 Kelvin.
Sirius.
Sirius is an A one star of absolute magnitude 1.43 at a distance of 81.4 Peta-Meters, and is the brightest star in the night sky.
Sirius B is a “D” “A” two dwarf, of absolute magnitude 11.33, gravitationally bound to Sirius “A”, at a distance of 3000 Giga-meters.
Sirius “A” has a Mass of 4.1 Ronna-Tons, a Radius of 1.19 Giga-Meters and a Temperature of 9,940K. The metallicity ratio is minus zero point 13 Dex. Sirius “A” is very young, with an age of 240 Mega years.
Due to its high luminosity, the inner edge of the habitable zone for Sirius “A” is around the same distance as from the Sun to Jupiter.
Sirius B has a Mass of 2.02 Ronna-Tons, a Radius 6 Mega-meters, and a Temperature of twenty five thousand kelvin. Whereas Sirius “A” is slightly larger than the Sun, the size of Sirius B is approximately equal to Earth. Sirius “B” is a young star, with an age of two hundred and thirty mega years.
Sirius “A” and “B” orbit around a common Barycenter, and the respective orbits can be seen quite readily from Earth.
At present the maximum mass of any planets in the Sirius system would have to be less than around fifteen times the mass of Jupiter.
Luyten “7 2 6” dash 8, also known as GJ 69, or Gliese 65.
Luyten “7 2 6” dash 8 “A” is an M five point 5 of absolute magnitude 15 point 27, located at 82.6 Peta meters from Earth.
Luyten “7 2 6” dash 8 “B” is an M five point 5 of absolute magnitude 16 point 11, at a separation of 2340 Giga-meters from Luyten “A”.
Luyten “7 2 6” dash 8 “B” was originally named UV Ceti, and is a UV Ceti flare star.
Luyten “7 2 6” dash 8 “A” is a BL Ceti type flare star, with a rotation period of 0.2 days.
Lutyen “7 2 6” dash 8 “A” has a LILO of 22.262, and a log ten GM of 19.13, Luyten “7 2 6” dash 8 B has a LILO of 21.086, Log ten GM 19.12. Since they are both small and dim, their habitable zones extend from one to one point five Giga-meters.
At present there are no known planets for this system.
Ross 154.
Ross 154 is an M four point 5 of absolute magnitude 13, at 91.7 Peta meters. Ross 154 is an active UV-Ceti type flare star, with the designation V1216 Sagitarri.
Light curve variations of up to 1.5 percent. Flares of up to 2.3 times 10 to the 26 Joules have been detected. There are significant x-ray components. It does not display any excess infrared absorption, which would indicate planetary companions.
Ross 154 has a Mass of 0.352 Ronna-tons, Radius of 0.139 Giga-meters, and a Temperature of 3,248 Kelvin. Its Metallicity is minus 0.25, and it is around zero point seven Giga-years old.
Ross 154 has a rotation rate of 2.85 days.
Ross 248.
Ross 248 is an M four point 6 of absolute magnitude 14 point 79, located at a distance 97 point 7 Peta meters. Due to its high- relative velocity Ross 248 will be the closest star to earth in 36,000 years time. Ross 248 is a B, Y Draconis type variable star, with the designation H, H Andromedae. B, Y Draconis variables have quasi-periodic light curves due to rotation and active chromospheres.
Ross 248 has a Mass of 0.288 Ronna-tons, Radius 0.132 Giga-meters, and a Temperature 2930 Kelvisn. Its Metallicity is 0.23, and its age is 2.6 Giga-years. Ross 248 has a rotation period of 99.58 days.
Epsilon Eridani.
Epsilon Eridani is a K two of absolute magnitude 6 point 18, and is located at a distance of 99.3 Peta meters.
Epsilon Eridani has a rotation period of 11.4 days and is a B Y Draconis variable star. It is around six hundred million years old. The X-ray luminosity of Epsilon Eridani is 2 times ten to the 21 Watts.
The metallicity of Epsilon Eridani is minus zero point one three.
Epsilon Eridani is 4 to 8 hundred million years old, and has a rotational period of 11.4 days
Epsilon Eridani is a very dusty star, with significant infrared absorption indicating an asteroid belt at 200 to 600 Giga-meters, another asteroid belt at 1,000 to 3 thousand Giga-meters, and a dust disk extending out to 15 Tera-meters.
It has one confirmed planet Epsilon Eridani b, with a mass of 0.78 of Jupiter.
Epsilon Eridani b’s parameters are eccentricity 0.07, period 7.37 years, with a semi major axis of 521 Giga-meters.
Lacaille 9352, Also known as GJ887.
Lacaille 9352 is an M zero point five of absolute magnitude 9 point 76, at 101 point 5 Peta meters from Earth.
Lacaille 9352 was the first red-dwarf to have its diameter measured.
Lacaille 9352 is 4.57 Giga-years old, with an Iron to Hydrogen Metallicity of minus 0.06, and a temperature of 3688 K.
The mass of Lacaille 9352 is 0.952 Ronna-tons, and its diameter is 0.330 Giga-meters.
At least two planets are reported for this system, at 10.1 Giga-meters mass greater than four times earth, and 17.9 Giga-meters, mass greater than seven times Earth. Lacaille 9352 is a suspected variable star, although with no observation evidence of variability.
GJ887b has a mass of 4 earths, a period of 9.262 days, an eccentricity of 0.09, and a semi major axis of 10.3 Giga-meters. Its equilibrium temperature would be 468 Kelvin.
GJ887c has a mass of 7.6 earths, a period of 21.789 days, an eccentricity of 0.22, and a semi major axis of 17.9 Giga-meters. Its equilibrium temperature would be 352Kelvin.
Ross 128.
Ross 128 is an M four dwarf, F 1 Virginis type flare star, with an absolute visual magnitude of 13.53.
The rotational period is 121 days. The flare activity is low compared to BY Draconis type flares, with an amplitude of a thousandth of a magnitude, mainly in the X-ray spectrum with very little UV or visible variation.
Ross 128 is 103 Peta-meters from Sol, Is 5 Giga-years old and has a temperature of 3192 K.
Ross 128 has a mass of 0.334 Ronna-tons, a diameter of 0.137 Giga-meters, and an Iron over Hydrogen metallicity of minus 0.02.
A confirmed rocky planet, Ross 128 b, is located at 7.4 Giga-meters, with an orbital period of about 9.866 Earth days. As the metallicity of Ross 128 is close to that of the sun, the dimensions of the planet are expected to be similar to Earth, with a similar surface temperature.
Orbital parameters for Ross 128 b are eccentricity 0.116, period 9.866 days, Semi major axis 7.42 Giga-meters. Ross 128 b has a mass of 1.8 of Earth, and an equilibrium temperature of 294 Kelvin.
Chahal 2022. Statistics of BY Draconis Chromospheric Variable Stars (https://arxiv.org/pdf/2206.05505.pdf)
Recalculating the Orbit of α-Centauri AB
https://arxiv.org/ftp/arxiv/papers/1007/1007.2293.pdf
Proxima's orbit around Alpha Centauri
https://arxiv.org/abs/1611.03495
Zhao 2017 PLANET DETECTABILITY IN THE ALPHA CENTAURI SYSTEM
https://arxiv.org/abs/1711.06320
Precision Millimeter Astrometry of the α Centauri AB System
https://arxiv.org/pdf/2104.10086.pdf
Breezing through the space environment of Barnard's Star b
https://arxiv.org/abs/1901.00219
Stellar Activity Manifesting at a One Year Alias Explains Barnard b as a False Positive
https://arxiv.org/abs/2105.07005
Searching for Planets Orbiting Alpha Centauri A with the James Webb Space Telescope
https://arxiv.org/abs/1910.09709
Toldeo 2018
Stellar activity analysis of Barnard's Star: Very slow rotation and evidence for long-term activity cycle
https://arxiv.org/abs/1812.06712v3
EDEN: Flare Activity of the Nearby Exoplanet-hosting M Dwarf Wolf 359 Based on K2 and EDEN Light Curves.
https://iopscience.iop.org/article/10.3847/1538-3881/abf933/pdf
The Astronomical Journal, 162:11 (14pp), 2021 July
Pineda 2021
The M-dwarf Ultraviolet Spectroscopic Sample I. Determining Stellar Parameters for Field Stars
https://arxiv.org/abs/2106.07656
Ribas 2023
The CARMENES search for exoplanets around M dwarfs. Guaranteed time observations Data Release 1 (2016-2020)
https://arxiv.org/abs/2302.10528
Stock 2020
The CARMENES search for exoplanets around M dwarfs. Three temperate to warm super-Earths
https://arxiv.org/abs/2010.00474
The Sirius System and Its Astrophysical Puzzles: Hubble Space Telescope and Ground-based Astrometry
The Astrophysical Journal, 840:70 (17pp), 2017 May 10
https://arxiv.org/abs/1703.10625
Wargelin 2007
X-Ray Flaring on the dMe Star, Ross 154
https://arxiv.org/abs/0712.2791
Brown 2023
Coronal X-Ray Emission from Nearby, Low-mass, Exoplanet Host Stars Observed by the MUSCLES and Mega-MUSCLES HST Treasury Survey Project
The Astronomical Journal, 165:195 (19pp), 2023 May
https://iopscience.iop.org/article/10.3847/1538-3881/acc38a/pdf
Santos 2003
Spectroscopic [Fe/H] for 98 extra-solar planet-host stars: Exploring the probability of planet formation
https://arxiv.org/abs/astro-ph/0311541
Mawet 2019
Deep Exploration of Epsilon Eridani with Keck Ms-band Vortex Coronagraphy and Radial Velocities: Mass and Orbital Parameters of the Giant Exoplanet.
The Astronomical Journal, 157:33 (20pp), 2019 January
https://arxiv.org/abs/1810.03794
Jeffers 2020
A multiple planet system of super-Earths orbiting the brightest red dwarf star GJ887
https://arxiv.org/abs/2006.16372
Bonfils 2017
A temperate exo-Earth around a quiet M dwarf at 3.4 parsecs
https://arxiv.org/abs/1711.06177
Wright 2017
Radial Velocities as an Exoplanet Discovery Method.
https://arxiv.org/pdf/1707.07983.pdf
Koch 2010
Kepler Mission Design, Realized Photometric Performance, and Early Science.
https://arxiv.org/ftp/arxiv/papers/1001/1001.0268.pdf
Bonnefoy 2018
The GJ 504 system revisited Combining interferometric, radial velocity, and high contrast imaging data
https://arxiv.org/pdf/1807.00657.pdf
-
2:08:45
Kim Iversen
11 hours agoNew Year, New PSYOP?: The Fort Bragg Connection In The New Years Terror Attacks
72.6K168 -
1:41:18
Glenn Greenwald
10 hours agoTerror Attacks Exploited To Push Unrelated Narratives; Facing Imminent Firing Squad, Liz Cheney Awarded Presidential Medal | SYSTEM UPDATE #381
103K183 -
1:00:32
Man in America
12 hours ago🔴 LIVE: Terror Attacks or False Flags? IT DOESN'T ADD UP!!!
69.2K24 -
1:02:38
Donald Trump Jr.
14 hours agoNew Year’s Terror, Latest Breaking News with Sebastian Gorka | TRIGGERED Ep.204
201K417 -
59:59
The StoneZONE with Roger Stone
9 hours agoAfter Years of Targeting Trump, FBI and DOJ are Unprepared to Stop Terror Attacks | The StoneZONE
65.6K28 -
1:26:42
Leonardaisfunny
8 hours ago $4.70 earnedH-1b Visas: Infinity Indians
43.1K25 -
1:08:33
Josh Pate's College Football Show
12 hours ago $2.75 earnedPlayoff Reaction Special: Ohio State Owns Oregon | Texas Survives | UGA vs Notre Dame Takeaways
39.6K6 -
58:04
Kimberly Guilfoyle
12 hours agoFBI's Terror Response Failures, Live with Steve Friend & Kyle Seraphin | Ep. 185
113K45 -
2:15:01
WeAreChange
13 hours agoMassive Developments In Vegas Investigation! UNREAL DETONATION, Shocking Details Emerge!
118K89 -
54:02
LFA TV
19 hours ago2025 Is Off to a Violent Start | TRUMPET DAILY 1.2.25 7pm
51.8K12