Premium Only Content
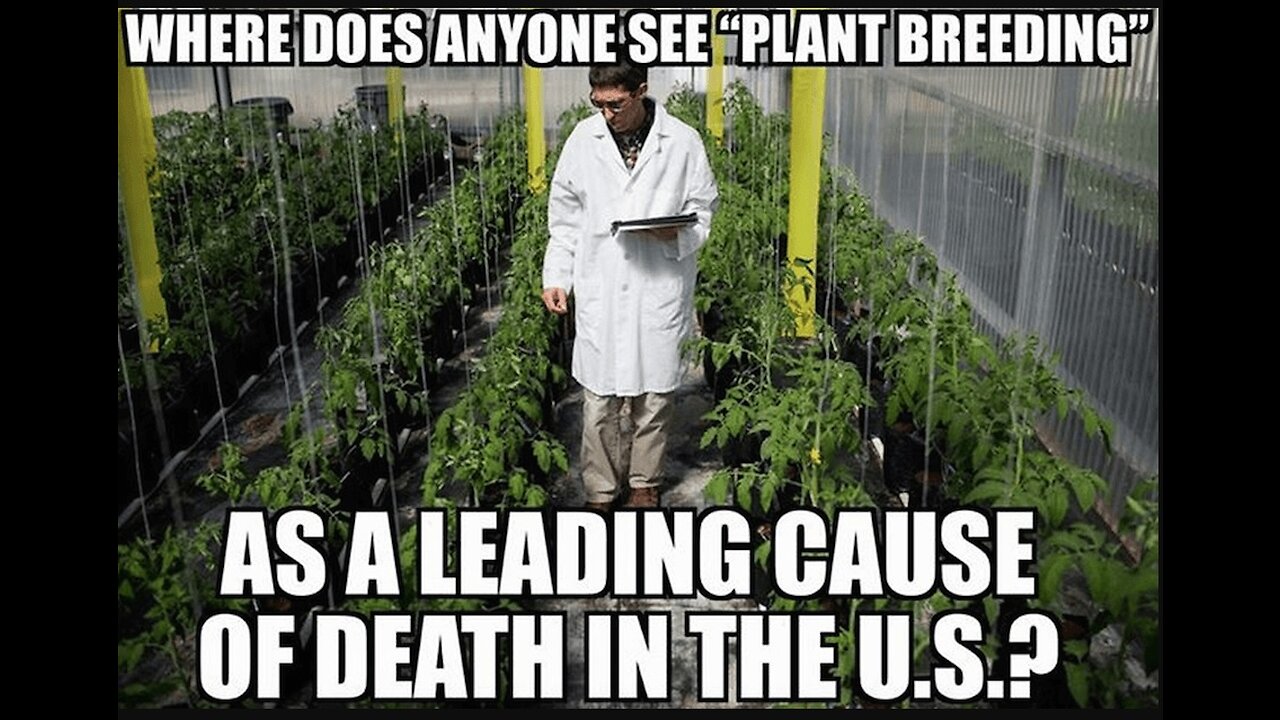
GMO Genetically Modified Organisms Transgenic Crops and Recombinant DNA Technology
Genetically Modified Organisms ? - So Poor Johnny doesn't like "healthy" food. What could possibly persuade him to eat all his "veggies"? If you could save lives by producing vaccines in transgenic bananas, would you? In the debate over large-scale commercialization and use of GMOs, where should we draw the line? ou may use this film Only if it stays intact. No part of it. may be removed or used in part without our permission.
People have been altering the genomes of plants and animals for many years using traditional breeding techniques. Artificial selection for specific, desired traits has resulted in a variety of different organisms, ranging from sweet corn to hairless cats. But this artificial selection, in which organisms that exhibit specific traits are chosen to breed subsequent generations, has been limited to naturally occurring variations. In recent decades, however, advances in the field of genetic engineering have allowed for precise control over the genetic changes introduced into an organism. Today, we can incorporate new genes from one species into a completely unrelated species through genetic engineering, optimizing agricultural performance or facilitating the production of valuable pharmaceutical substances. Crop plants, farm animals, and soil bacteria are some of the more prominent examples of organisms that have been subject to genetic engineering.
Current Use of Genetically Modified Organisms Agricultural plants are one of the most frequently cited examples of genetically modified organisms (GMOs). Some benefits of genetic engineering in agriculture are increased crop yields, reduced costs for food or drug production, reduced need for pesticides, enhanced nutrient composition and food quality, resistance to pests and disease, greater food security, and medical benefits to the world's growing population. Advances have also been made in developing crops that mature faster and tolerate aluminum, boron, salt, drought, frost, and other environmental stressors, allowing plants to grow in conditions where they might not otherwise flourish (Table 1; Takeda & Matsuoka, 2008). Other applications include the production of nonprotein (bioplastic) or nonindustrial (ornamental plant) products. A number of animals have also been genetically engineered to increase yield and decrease susceptibility to disease. For example, salmon have been engineered to grow larger (Figure 1) and mature faster (Table 1), and cattle have been enhanced to exhibit resistance to mad cow disease (United States Department of Energy, 2007).
The pharmaceutical industry is another frontier for the use of GMOs. In 1986, human growth hormone was the first protein pharmaceutical made in plants (Barta et al., 1986), and in 1989, the first antibody was produced (Hiatt et al., 1989). Both research groups used tobacco, which has since dominated the industry as the most intensively studied and utilized plant species for the expression of foreign genes (Ma et al., 2003). As of 2003, several types of antibodies produced in plants had made it to clinical trials. The use of genetically modified animals has also been indispensible in medical research. Transgenic animals are routinely bred to carry human genes, or mutations in specific genes, thus allowing the study of the progression and genetic determinants of various diseases.
Potential GMO Applications
Many industries stand to benefit from additional GMO research. For instance, a number of microorganisms are being considered as future clean fuel producers and biodegraders. In addition, genetically modified plants may someday be used to produce recombinant vaccines. In fact, the concept of an oral vaccine expressed in plants (fruits and vegetables) for direct consumption by individuals is being examined as a possible solution to the spread of disease in underdeveloped countries, one that would greatly reduce the costs associated with conducting large-scale vaccination campaigns. Work is currently underway to develop plant-derived vaccine candidates in potatoes and lettuce for hepatitis B virus (HBV), enterotoxigenic Escherichia coli (ETEC), and Norwalk virus. Scientists are also looking into the production of other commercially valuable proteins in plants, such as spider silk protein and polymers that are used in surgery or tissue replacement (Ma et al., 2003). Genetically modified animals have even been used to grow transplant tissues and human transplant organs, a concept called xenotransplantation. The rich variety of uses for GMOs provides a number of valuable benefits to humans, but many people also worry about potential risks.
Risks and Controversies Surrounding the Use of GMOs
Despite the fact that the genes being transferred occur naturally in other species, there are unknown consequences to altering the natural state of an organism through foreign gene expression. After all, such alterations can change the organism's metabolism, growth rate, and/or response to external environmental factors. These consequences influence not only the GMO itself, but also the natural environment in which that organism is allowed to proliferate. Potential health risks to humans include the possibility of exposure to new allergens in genetically modified foods, as well as the transfer of antibiotic-resistant genes to gut flora.
Horizontal gene transfer of pesticide, herbicide, or antibiotic resistance to other organisms would not only put humans at risk, but it would also cause ecological imbalances, allowing previously innocuous plants to grow uncontrolled, thus promoting the spread of disease among both plants and animals. Although the possibility of horizontal gene transfer between GMOs and other organisms cannot be denied, in reality, this risk is considered to be quite low. Horizontal gene transfer occurs naturally at a very low rate and, in most cases, cannot be simulated in an optimized laboratory environment without active modification of the target genome to increase susceptibility (Ma et al., 2003).
In contrast, the alarming consequences of vertical gene transfer between GMOs and their wild-type counterparts have been highlighted by studying transgenic fish released into wild populations of the same species (Muir & Howard, 1999). The enhanced mating advantages of the genetically modified fish led to a reduction in the viability of their offspring. Thus, when a new transgene is introduced into a wild fish population, it propagates and may eventually threaten the viability of both the wild-type and the genetically modified organisms.
Unintended Impacts on Other Species: The Bt Corn Controversy
One example of public debate over the use of a genetically modified plant involves the case of Bt corn. Bt corn expresses a protein from the bacterium Bacillus thuringiensis. Prior to construction of the recombinant corn, the protein had long been known to be toxic to a number of pestiferous insects, including the monarch caterpillar, and it had been successfully used as an environmentally friendly insecticide for several years. The benefit of the expression of this protein by corn plants is a reduction in the amount of insecticide that farmers must apply to their crops. Unfortunately, seeds containing genes for recombinant proteins can cause unintentional spread of recombinant genes or exposure of non-target organisms to new toxic compounds in the environment.
The now-famous Bt corn controversy started with a laboratory study by Losey et al. (1999) in which the mortality of monarch larvae was reportedly higher when fed with milkweed (their natural food supply) covered in pollen from transgenic corn than when fed milkweed covered with pollen from regular corn. The report by Losey et al. was followed by another publication (Jesse & Obrycki, 2000) suggesting that natural levels of Bt corn pollen in the field were harmful to monarchs.
Debate ensued when scientists from other laboratories disputed the study, citing the extremely high concentration of pollen used in the laboratory study as unrealistic, and concluding that migratory patterns of monarchs do not place them in the vicinity of corn during the time it sheds pollen. For the next two years, six teams of researchers from government, academia, and industry investigated the issue and concluded that the risk of Bt corn to monarchs was "very low" (Sears et al., 2001), providing the basis for the U.S. Environmental Protection Agency to approve Bt corn for an additional seven years.
Unintended Economic Consequences
Another concern associated with GMOs is that private companies will claim ownership of the organisms they create and not share them at a reasonable cost with the public. If these claims are correct, it is argued that use of genetically modified crops will hurt the economy and environment, because monoculture practices by large-scale farm production centers (who can afford the costly seeds) will dominate over the diversity contributed by small farmers who can't afford the technology. However, a recent meta-analysis of 15 studies reveals that, on average, two-thirds of the benefits of first-generation genetically modified crops are shared downstream, whereas only one-third accrues upstream (Demont et al., 2007). These benefit shares are exhibited in both industrial and developing countries. Therefore, the argument that private companies will not share ownership of GMOs is not supported by evidence from first-generation genetically modified crops.
GMOs and the General Public: Philosophical and Religious Concerns
In a 2007 survey of 1,000 American adults conducted by the International Food Information Council (IFIC), 33% of respondents believed that biotech food products would benefit them or their families, but 23% of respondents did not know biotech foods had already reached the market. In addition, only 5% of those polled said they would take action by altering their purchasing habits as a result of concerns associated with using biotech products.
According to the Food and Agriculture Organization of the United Nations, public acceptance trends in Europe and Asia are mixed depending on the country and current mood at the time of the survey (Hoban, 2004). Attitudes toward cloning, biotechnology, and genetically modified products differ depending upon people's level of education and interpretations of what each of these terms mean. Support varies for different types of biotechnology; however, it is consistently lower when animals are mentioned.
Furthermore, even if the technologies are shared fairly, there are people who would still resist consumable GMOs, even with thorough testing for safety, because of personal or religious beliefs. The ethical issues surrounding GMOs include debate over our right to "play God," as well as the introduction of foreign material into foods that are abstained from for religious reasons. Some people believe that tampering with nature is intrinsically wrong, and others maintain that inserting plant genes in animals, or vice versa, is immoral. When it comes to genetically modified foods, those who feel strongly that the development of GMOs is against nature or religion have called for clear labeling rules so they can make informed selections when choosing which items to purchase. Respect for consumer choice and assumed risk is as important as having safeguards to prevent mixing of genetically modified products with non-genetically modified foods. In order to determine the requirements for such safeguards, there must be a definitive assessment of what constitutes a GMO and universal agreement on how products should be labeled.
These issues are increasingly important to consider as the number of GMOs continues to increase due to improved laboratory techniques and tools for sequencing whole genomes, better processes for cloning and transferring genes, and improved understanding of gene expression systems. Thus, legislative practices that regulate this research have to keep pace. Prior to permitting commercial use of GMOs, governments perform risk assessments to determine the possible consequences of their use, but difficulties in estimating the impact of commercial GMO use makes regulation of these organisms a challenge.
History of International Regulations for GMO Research and Development
In 1971, the first debate over the risks to humans of exposure to GMOs began when a common intestinal microorganism, E. coli, was infected with DNA from a tumor-inducing virus (Devos et al., 2007). Initially, safety issues were a concern to individuals working in laboratories with GMOs, as well as nearby residents. However, later debate arose over concerns that recombinant organisms might be used as weapons. The growing debate, initially restricted to scientists, eventually spread to the public, and in 1974, the National Institutes of Health (NIH) established the Recombinant DNA Advisory Committee to begin to address some of these issues.
In the 1980s, when deliberate releases of GMOs to the environment were beginning to occur, the U.S. had very few regulations in place. Adherence to the guidelines provided by the NIH was voluntary for industry. Also during the 1980s, the use of transgenic plants was becoming a valuable endeavor for production of new pharmaceuticals, and individual companies, institutions, and whole countries were beginning to view biotechnology as a lucrative means of making money (Devos et al., 2007). Worldwide commercialization of biotech products sparked new debate over the patentability of living organisms, the adverse effects of exposure to recombinant proteins, confidentiality issues, the morality and credibility of scientists, the role of government in regulating science, and other issues. In the U.S., the Congressional Office of Technology Assessment initiatives were developed, and they were eventually adopted worldwide as a top-down approach to advising policymakers by forecasting the societal impacts of GMOs.
Then, in 1986, a publication by the Organization for Economic Cooperation and Development (OECD), called "Recombinant DNA Safety Considerations," became the first intergovernmental document to address issues surrounding the use of GMOs. This document recommended that risk assessments be performed on a case-by-case basis. Since then, the case-by-case approach to risk assessment for genetically modified products has been widely accepted; however, the U.S. has generally taken a product-based approach to assessment, whereas the European approach is more process based (Devos et al., 2007). Although in the past, thorough regulation was lacking in many countries, governments worldwide are now meeting the demands of the public and implementing stricter testing and labeling requirements for genetically modified crops.
Increased Research and Improved Safety Go Hand in Hand
Proponents of the use of GMOs believe that, with adequate research, these organisms can be safely commercialized. There are many experimental variations for expression and control of engineered genes that can be applied to minimize potential risks. Some of these practices are already necessary as a result of new legislation, such as avoiding superfluous DNA transfer (vector sequences) and replacing selectable marker genes commonly used in the lab (antibiotic resistance) with innocuous plant-derived markers (Ma et al., 2003). Issues such as the risk of vaccine-expressing plants being mixed in with normal foodstuffs might be overcome by having built-in identification factors, such as pigmentation, that facilitate monitoring and separation of genetically modified products from non-GMOs. Other built-in control techniques include having inducible promoters (e.g., induced by stress, chemicals, etc.), geographic isolation, using male-sterile plants, and separate growing seasons.
GMOs benefit mankind when used for purposes such as increasing the availability and quality of food and medical care, and contributing to a cleaner environment. If used wisely, they could result in an improved economy without doing more harm than good, and they could also make the most of their potential to alleviate hunger and disease worldwide. However, the full potential of GMOs cannot be realized without due diligence and thorough attention to the risks associated with each new GMO on a case-by-case basis.
GMO toxicity: fears and scientific analysis
After genetically modified foods were introduced in the United States a few decades ago, people independently reported toxic effects caused by GMOs. One example is an anti-GMO advocacy group called the Institute for Responsible Technology (IRT), which reported that rats fed a diet containing a GMO potato had virtually every organ system adversely affected after just ten days of feeding. The IRT stated that the toxicity was the result of genetic modification techniques and not a specific case for that particular potato. They claimed the process of making the GMO caused it to be toxic and thus all GMOs were high risk for toxicity.
Scientists across the U.S. and the rest of the world have sought to rigorously test the assertions of the IRT and others to uncover any possible toxicity caused by GMOs. To this end, many different types of modifications in various crops have been tested, and the studies have found no evidence that GMOs cause organ toxicity or other adverse health effects. An example of this research is a study carried out on a type of GMO potato that was genetically modified to contain the bar gene. The product of the bar gene is an enzyme that can detoxify herbicides and thus protects the potato from herbicidal treatment.
In order to see if this GMO potato would have adverse effects on consumer health like those claimed by the IRT, a group of scientists at the National Institute of Toxicological Research in Seoul, Korea fed rats diets containing either GMO potato or non-GMO potato. For each diet, they tracked male and female rats. To carefully analyze the rats’ health, a histopathological examination of tissues and organs was conducted after the rats died. Histopathology is the examination of organs for disease at the microscopic level (think pathologist doing a biopsy). Histopathological examinations of the reproductive organs, liver, kidneys, and spleen showed no differences between GMO-eating and non-GMO-eating animals.
Nobody has ever been killed by exposure to gamma rays from a cobalt-60 source used in food irradiation. But people die all the time from food poisoning that is preventable by this technology. We just need to put our worries in the proper perspective. One anti-irradiation advocate was heard to say: “When I shop, I seek mold as a confirmation that the food I am buying is not irradiated.” What silliness.
Food irradiation is a technology used to improve the safety and extend the shelf life of foods by reducing or eliminating microorganisms and insects. It is considered a “cold” method, meaning it doesn’t use heat to kill germs, unlike pasteurization and canning which use heat. Food irradiation works by exposing food products to ionizing radiation, making them safer for the consumer.
Irradiation does not make foods radioactive, compromise nutritional quality, or noticeably change the taste, texture, or appearance of food. In fact, any changes made by irradiation are so minimal that it is not easy to tell if a food has been irradiated.
Food irradiation (the application of ionizing radiation to food) is a technology that improves the safety and extends the shelf life of foods by reducing or eliminating microorganisms and insects. Like pasteurizing milk and canning fruits and vegetables, irradiation can make food safer for the consumer. The Food and Drug Administration (FDA) is responsible for regulating the sources of radiation that are used to irradiate food. The FDA approves a source of radiation for use on foods only after it has determined that irradiating the food is safe.
Irradiation can serve many purposes.
Prevention of Foodborne Illness – to effectively eliminate organisms that cause foodborne illness, such as Salmonella and Escherichia coli (E. coli).
Preservation – to destroy or inactivate organisms that cause spoilage and decomposition and extend the shelf life of foods.
Control of Insects – to destroy insects in or on tropical fruits imported into the United States. Irradiation also decreases the need for other pest-control practices that may harm the fruit.
Delay of Sprouting and Ripening – to inhibit sprouting (e.g., potatoes) and delay ripening of fruit to increase longevity.
Sterilization – irradiation can be used to sterilize foods, which can then be stored for years without refrigeration. Sterilized foods are useful in hospitals for patients with severely impaired immune systems, such as patients with AIDS or Covid 19 shot's and or undergoing chemotherapy. Foods that are sterilized by irradiation are exposed to substantially higher levels of treatment than those approved for general use.
There are three sources of radiation approved for use on foods.
Gamma rays are emitted from radioactive forms of the element cobalt (Cobalt 60) or of the element cesium (Cesium 137). Gamma radiation is used routinely to sterilize medical, dental, and household products and is also used for the radiation treatment of cancer.
X-rays are produced by reflecting a high-energy stream of electrons off a target substance (usually one of the heavy metals) into food. X-rays are also widely used in medicine and industry to produce images of internal structures.
Electron beam (or e-beam) is similar to X-rays and is a stream of high-energy electrons propelled from an electron accelerator into food.
The FDA has evaluated the safety of irradiated food for more than 30 years and has found the process to be safe. The World Health Organization (WHO), the Centers for Disease Control and Prevention (CDC) and the U.S. Department of Agriculture (USDA) have also endorsed the safety of irradiated food.
The FDA has approved a variety of foods for irradiation in the United States including: Beef and Pork - Crustaceans (e.g., lobster, shrimp, and crab) - Fresh Fruits and Vegetables - Lettuce and Spinach - Poultry - Seeds for Sprouting (e.g., for alfalfa sprouts) - Shell Eggs - Shellfish - Molluscan - (e.g., oysters, clams, mussels, and scallops) - Spices and Seasonings.
The FDA requires that irradiated foods bear the international symbol for irradiation. Look for the Radura symbol along with the statement “Treated with radiation” or “Treated by irradiation” on the food label. Bulk foods, such as fruits and vegetables, are required to be individually labeled or to have a label next to the sale container. The FDA does not require that individual ingredients in multi-ingredient foods (e.g., spices) be labeled. It is important to remember that irradiation is not a replacement for proper food handling practices by producers, processors, and consumers. Irradiated foods need to be stored, handled, and cooked in the same way as non-irradiated foods, because they could still become contaminated with disease-causing organisms after irradiation if the rules of basic food safety are not followed.
irradiated food will kill you ? Irradiation is used to reduce or remove pathogens, such as bacteria and molds, that spoil food and cause food poisoning and other illness. It works by applying radiant energy waves to the food which absorbs energy and kills the bacteria that can cause food poisoning in a similar way that heat energy kills bacteria when food is cooked.
Is Food Irradiation Dangerous?
Try asking people what they are more scared of, exposing food to nuclear radiation or eating food contaminated by E. coli, Listeria or Salmonella bacteria. It’s a safe bet that many would rather take their chances with bacterial food poisoning than with food irradiation. Too bad because food irradiation can reduce the risk of bacterial food poisoning, but public fear keeps the technology from being more widely applied than it currently is. Try asking people what they are more scared of, exposing food to nuclear radiation or eating food contaminated by E. coli, Listeria or Salmonella bacteria. It’s a safe bet that many would rather take their chances with bacterial food poisoning than with food irradiation. Too bad because food irradiation can reduce the risk of bacterial food poisoning, but public fear keeps the technology from being more widely applied than it currently is. And this fear is mostly irrational. The idea behind food irradiation is to destroy bacteria by exposing them to free radicals which are highly reactive and can interrupt cell division. The needed free radicals are generated by bombarding the food with gamma rays released from a cobalt-60 source. This does not make the food radioactive or dangerous in any way. Food irradiation technology has been around a longtime and today most of the spices, herbs and dried vegetables we import are treated in this fashion without any problem ever having appeared. The technique can be used to prevent sprouting in potatoes, extend the shelf life of strawberries and reduce insect infestation in mangoes and papayas.
The real potential benefit, though, would be in treating fresh and frozen meat to reduce the risk of Salmonella, E. coli and Listeria poisoning. The latter is a serious concern with ready- to-eat meat products such as sausages, luncheon meats and cooked ham. In any case, anyone desiring to avoid irradiated foods could easily do so because by law such foods have to be labeled with the symbol known as the radura. Some anti-irradiation activists claim that exposing foods to gamma rays destroys nutrients and gives rise to what they call unique “radiolytic products” of unknown toxicity. Gamma rays from cobalt-60 can destroy some of the A,C,E, and B vitamins but under the conditions used this effect is very small. True, on exposure to irradiation chemical bonds are broken and new molecules can form. Most have not been identified and tested but formaldehyde does form in irradiated starch, benzene in meat, peroxides in plant tissues and formic acid in sucrose. All such radiolytic products total no more than 30 ppm. There is more benzene in non-irradiated dairy products than in meat that has been irradiated. Countries such as Israel use irradiation on a large scale and no problems have been noted. In fact, some studies have shown that irradiation increases the concentration of some beneficial substances such as flavanols in citrus fruits. The one realistic concern with food irradiation concerns the cobalt-60 source. This has to be transported to the irradiation facility and in theory accidents can happen along the way. But current methods of transporting radioactive materials are very sophisticated and accidental release of radiation is extremely unlikely.
Nobody has ever been killed by exposure to gamma rays from a cobalt-60 source used in food irradiation. But people die all the time from food poisoning that is preventable by this technology. We just need to put our worries in the proper perspective. One anti-irradiation advocate was heard to say: “When I shop, I seek mold as a confirmation that the food I am buying is not irradiated.” What silliness. Needless to say, some molds are highly toxic.
Genetically modified organism (GMO) patents refer to intellectual property rights that the United States government issues to inventors of GMOs as a form of protection against infringement. GMOs are food products that have been artificially modified through genetic engineering to possess new traits and benefits.
We currently live in the dawn of the age of modern biotechnology which has delivered many benefits to various industries, including that of the agricultural sector. Some of these benefits, which are passed onto society as a whole, have come thanks to companies being able to protect their intellectual property via patents, including genetically-modified organisms (GMO’s). However, there has been an aggressive push from science denialists from the anti-GMO camp to demonize this technology, which has combined with those who are against intellectual property rights and patent laws. This article examines the background to agribiotech and patent protection, arguments for and against GMO patents, and a final reasoning as to why the latter group are incorrect in their anti-science position.
Introduction
Humans have been engaging in biotechnology (that is, the use of living organisms and manipulation of their genes to produce goods) since the dawn of civilization; just look at the exploitation of yeast for the fermentation process to produce beer in ancient Sumeria and Babylonia in ~7000 BCE (Colwell, 2017). However, it wasn’t until 1972, when Herbert Boyer and Stanley Cohen first transferred DNA from one microorganism to another, that we found ourselves in the dawn of modern biotechnology (Cohen, et al., 1973). In contrast to the past, we now have a plethora of sophisticated and precise tools (such as the now famous method known as CRISPR) at our disposal to manipulate the genes of organisms to develop desired products (Gao, 2018). However, these tools have not come cheap, and are in fact the fruits of years of research and an enormous injection of capital. In fact, in the United States, it takes, on average, 8 years and $136 million to develop a new genetically-modified (GM) product for agricultural use, including getting through the intense regulatory hurdles (McDougall, 2011). Due to this significant investment, scientists, universities, and companies need to ensure staunch protection around their intellectual property (IP), and the most common method involves the use of patents.
Patents, as defined by the World Intellectual Property Organisation (WIPO), are ‘an exclusive right granted for an invention, which is a product or a process that provides, in general, a new way of doing something, or offers a new technical solution to a problem’ (WIPO, n.d.). They afford the owners of a granted patent the right to exclude others from exploiting their IP for a period of, most commonly, 20 years. (Gwartney, 2009). This creates a temporary monopoly where the inventors have a chance (not a guarantee) to recoup expenses associated with the R&D that has gone into the development of a patented invention, without worrying about unfair competition (Clark, 2011). This is particularly important in the biomedical and biotechnological fields given the exceptionally high R&D costs associated with innovating. In fact, contrary to popular myth, patents in these fields do not hinder innovation, but rather facilitate it thanks to the use of licensing agreements, research exemptions from patent protections, and competitors being able to see the publicly available publish patent to then attempt to jump ahead to the next step in development (Clark, 2011). This article will present common arguments used both in favour of and against patenting genetically-modified organisms (GMO’s) in the agribiotech industry. Finally, the myths, misconceptions, and lies utilized by the anti-GMO and patent-skeptic community will be debunked through an evidence-based approach.
Debunking the Misconceptions & Solidifying the Case for GMO Patent Rights
Firstly, from a scientific perspective, one needs to merely look at the stark contrast in the type of references making claims about GMO’s and patents, with those against typically appearing in mere newspapers, blogs, and biased non-governmental organizations, rather than quality, peer-reviewed scientific and legal literature. Regarding the potential for environmental risk regarding the release of GMO’s, they are extensively tested with none causing problems, and also there is the worse risk of not using GMO crops to tackle food issues (Hall, 2016). And in terms of potential for future harm, well that applies to non-GMO’s too, but as study after study mounts showing the safety of GMO’s, the likelihood of an issue arising nears zero (Skeptical, 2015). One of the silliest arguments against GMO’s is the naturalistic fallacy, which is a logically fallacious argument; remember, smallpox is natural, floods are natural: nature simply does not care (Daston, 2015). It should also go without saying, that religious arguments are not to be taken seriously when we have a more rigorous system for understanding the world; science (Haggarty-Weir, 2018). Science and the scientific method are the best tools we have for exploring our understanding of the world around us and how things work. So any scientific issues should be dealt with scientifically, and not through delusion (aka, religion).
In terms of the anti-patent arguments, the Supreme Court decision in 2013 has often been misrepresented; in fact synthetic genes are indeed patentable (Klusty & Weinmeyer, 2015). Also there were numerous issues with the reasoning used in the Myriad genetics case, but that’s still legally debatable. Next, when people bring up the terminator technology they are being either disingenuous or are ignorant, since the terminator technology has never actually been commercially used (Monsanto, 2017). Further, this is an issue of contracts law, not patent law (Scott, 2017). The argument of patents stifling innovation is also something that has been thoroughly debunked, and interested readers are encouraged to check out Clark, 2011 (see figure below) and Zhou, 2015. Finally, the argument that the patent litigation system favors big business due to the requisite costs is simply not GMO patent-specific and regards legal system reform (Gyles, 2014). I do agree that we should be aiming to equal legal playing fields, but that applies to all areas of society.
Conclusions - Exploiting patents for GMO’s helps human society significantly advance, especially in the agricultural sector. It is imperative that we address the issue of GMO’s through the lens of science. That is to say, evidence over ideology. Otherwise we all risk losing out.
-
13:12
What If Everything You Were Taught Was A Lie?
19 days agoA Guide To The Different Types Of Calendars Old & New Used Around The World Today
3.89K3 -
6:01
Dr. Nick Zyrowski
22 hours agoIs Beef Tallow healthy? Here Is How I Use It...
37.4K13 -
19:54
Degenerate Jay
19 hours ago $2.68 earnedThe Decline Of Assassin's Creed's World
37.7K3 -
8:30
Chrissy Clark
13 hours agoBehind The Scenes Of Trump’s Inauguration🇺🇸 I Vlog
31.7K7 -
1:06:05
PMG
13 hours ago $3.80 earned"What the FDA is Hiding About Nicotine Will SHOCK You w/ Dr. Ardis"
17.4K9 -
23:15
The Based Mother
13 hours ago $1.57 earnedHOLY BLASPHEMY! Trump vs. Episcopal Bishop Budde at the National Cathedral in DC
12.4K47 -
14:20
RealitySurvival
12 hours agoDrug Cartels Designated As Foreign Terrorist Organizations
25.1K9 -
3:16:13
The Nunn Report - w/ Dan Nunn
1 day ago[Ep 591] Shock & Awe! Trump is Back! | Guest: Sam Anthony of [Your]News
40.9K8 -
3:21:30
CharLee Simons Presents Do Not Talk
1 day agoDO NOT TALK with SIMONE ANDERSON, & MIKE COOK (World Collapse. Are You Ready?)
22.4K10 -
1:38:35
FreshandFit
12 hours agoDecoding Lily Phillips' Lies Through An RP Lense!
84.7K32